How does astronomy use the electromagnetic spectrum?
There is more to light than meets the eye, and it teaches us a lot about the universe.
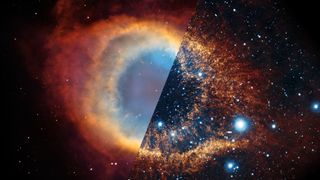
Parts of the electromagnetic spectrum invisible to human eyes reveal a vast amount of information about the universe, but it took a long time for astronomers to learn how to view it.
For thousands of years, humans were looking up at the star-studded night sky using just their eyes sensitive to the optical wavelength of the electromagnetic spectrum. The first telescopes, invented in the early 17th century, enhanced the ability of human eyes by magnifying distant objects.
But as physicists started discovering in the 19th century that there are other, invisible, types of light in the natural world around us, astronomers realized that there must be such light emanating also from the universe.
Related: Astronomy: The oldest scientific discipline
Today, astronomers know that the majority of radiation, or light, present in the universe is invisible to human eyes. By looking at the universe in all possible wavelengths, scientists are piecing together a complex picture of the unfathomably vast cosmic environment that we are a part of. It took, however, decades, for instruments to be developed that could detect this invisible radiation from celestial sources.
Here we explain what different parts of the electromagnetic spectrum teach us about the universe.
What do radio waves teach us about the universe?
Radio astronomy studies cosmic radiation with the longest wavelengths (from less than 0.4 inches to several miles, or 1 centimeter to several kilometers) and was the first kind of astronomy developed that relies on wavelengths other than optical light.
The discovery that radio waves from bodies in the universe lash our planet was made completely by accident. In 1933, a young American radio engineer Karl Jansky, an employee of the famous telephone company Bell Laboratories, was tasked to search for sources of unexplained hiss that sometimes interfered with transmissions of radio messages across the Atlantic Ocean. Jansky found that while some of this noise was coming from sources on Earth, such as nearby thunderstorms, there was a type of signal, constantly picked up by his experimental antennas, that appeared to be coming from what we know today is the center of our Milky Way galaxy, the region where the black hole Sagittarius A* resides. Systematic exploration of the radio universe began soon thereafter.
Astronomers have discovered since that radio waves are emitted by spinning electrons and emanate from all sorts of environments that have the ability to make those electrons spin, Affelia Wibisono, an astronomer at the Royal Observatory Greenwich in the U.K., told Space.com.
"Typically, when you detect radio waves, you're looking at electrons moving through a magnetic field," Wibisono said. "But ionized gas can emit radio waves as well."
By tracing the structure of radio wave-emitting clouds, astronomers were able to map out the entire structure of our galaxy, the Milky Way, as well as other nearby galaxies. They could determine areas with high concentrations of hot young stars, but also study objects obscured by dust, such as black holes that hide in galactic centers. Highly magnetized bodies, such as fast-spinning stellar remnants called pulsars are prime targets for radio astronomy as they send out powerful flashes of radio waves as they spin like superfast cosmic lighthouses.
Famous radio telescopes
As radio waves are the type of electromagnetic radiation with the longest wavelengths, radio telescopes have to be rather large. Vast arrays of radio-antennas, such as the Karl G. Jansky Very Large Array in New Mexico that consists of 28 dishes each 82-foot-wide (25 meters), are the technological standard today. By combining multiple antennas, astronomers create telescopes that have immense apertures that equal the distance between the array's most distant parts, thus enabling the scientist to detect the faintest signals with the best possible resolution.
The Square Kilometer Array (SKA), currently constructed across two locations in Australia and South Africa, will be the world's largest radio telescope by a significant margin once it comes online around 2028. With its thousands of dishes and dipole antennas spanning thousands of square miles of remote land, SKA will survey large areas of the sky at once and detect the faintest signals coming from the farthest reaches of the universe.
The Event Horizon Telescope, famous for taking photographs of black holes, is also a radio telescope, or rather a worldwide network of radio telescopes with an aperture equalling the size of our planet.
Unlike some other types of wavelengths, radio waves mostly penetrate Earth's atmosphere with ease, allowing astronomers to base their equipment on the planet's surface.
However, due to the ubiquity of radio communication technologies in the modern world, radio telescopes are at risk of getting confused by human-made signals. SKA, for example, will therefore be surrounded by a radio-quiet zone where no cell phones and no radio equipment will be allowed.
Constantly searching for better ways to study the universe, astronomers are now seriously considering building a radio telescope on the far side of the moon. Removed from Earth-based sources of human-made radio noise, as well as from Earth's ionosphere (the upper part of the atmosphere which contains ionized gas that absorbs and distorts some cosmic radio signals), such an observatory would provide scientists with the deepest and most undisturbed views into the earliest epoch of the universe.
What do microwaves teach us about the universe?
The next electromagnetic spectrum band after radio waves are microwaves. As microwaves cover wavelengths between 3.3 feet and 0.04 inches (1 meter and 1 millimeter), the first discoveries of cosmic microwaves were actually made by radio telescopes.
Microwaves have a special, although rather limited place in astronomy. According to the European Space Agency (ESA), the whole sky glows uniformly in microwaves with what has been identified as the cosmic microwave background.
This uniformness is unseen in other wavelengths, which reveal the sky in dots and regions of varying brightness. In fact, cosmic microwave radiation is so odd that the researchers who first discovered it in the 1960s (completely by accident during experiments with echo balloons) originally thought it was produced by a telescope defect.
Subsequent research, however, confirmed that the microwave hum was coming from space and that it was nothing less than the residue of radiation released by the Big Bang, the enormous explosion which created the universe some 13.8 billion years ago.
This radiation was originally released in the form of highly energetic, short-wavelength X-rays, but since it took so long to reach us, the so-called redshift effect caused by the expansion of the universe has stretched this wavelength all the way into microwaves.
Microwaves reveal the universe as it looked in its earliest stages. The most sensitive surveys were able to go as far as distinguishing the denser regions of gas and dust that subsequently produced the first galaxies.
Famous microwave telescopes
Microwaves get mostly absorbed by Earth's atmosphere, which means they are best studied by space-based telescopes.
In 1989, after the initial crude Earth-based detections of cosmic microwaves, NASA sent the first dedicated microwave-observing satellite — the Cosmic Background Explorer (COBE) — into space. COBE measured differences in the temperature of the microwave background in various regions. COBE's successor, the Wilkinson Microwave Anisotropy Probe (WMAP), launched in 2003, further improved the level of detail of this cosmic microwave map. These observations helped to determine the universe's age with greater precision, according to the European Space Agency (ESA), and define the amounts of different types of matter that the universe contained in its earliest years.
ESA's Planck mission, launched in 2009, then completed the task of creating the most accurate map of the cosmic microwave background, which, ESA said, is to some extent "definitive," as some of the measurements cannot be further improved.
What do submillimeter waves teach us about the universe?
The submillimeter wavelength sits between the millimeter and infrared ranges. As the name suggests, submillimeter waves have lengths shorter than 1 mm, or 0.04 inches, and up to a few hundred micrometers. Observations in this range partially overlap with the longest wavelengths of the infrared spectrum.
The use of submillimeter wavelengths in astronomy is relatively recent, according to Astronomy Cast. Detectors used to detect submillimeter radiation are quite similar to those used in radio astronomy, but thousands of times smaller. Technology therefore had to progress enough to make these detectors possible.
Speaking to the Astronomy Cast, an astronomy podcast, American astronomer Pamela Gay said that the use of submillimeter waves in astronomy is limited to certain types of objects and phenomena.
Submillimeter waves penetrate through clouds of molecular gas and dust into star-forming regions, which are obscured from the view of of optical telescopes.
In submillimeter waves, astronomers can observe universe's "natural lasers," regions where highly charged electrons emit laser light as they discharge some of their energy, said Gay. These natural lasers, sometimes called masers, are usually observed in a special type of pulsating variable stars called the Mira stars.
Submillimeter waves are also good at pointing astronomers to some interesting types of organic molecules and do a good job analyzing cold objects such as comets in the solar system, said Gay.
Famous submilimeter telescopes
Because submillimeter waves get absorbed by water in Earth's atmosphere, observatories that study sources of submillimeter radiation in the universe need to be built in high and dry places to prevent water vapor from obscuring their views. In essence, you will find submillimeter telescopes in the same places on Earth where you find the best optical telescopes.
The Atacama Large Millimeter/submillimeter Array (ALMA), operated by the European Southern Observatory is located on the Chajnantor plateau in northern Chile at an altitude of 16,400 feet (5,000 m).
The Submillimeter Array on Hawai'i's Maunakea, which is operated by the Harvard Smithsonian Center for Astrophysics, sits somewhat lower, at 13,450 feet (4,100 m) above sea level.
What does infrared light teach us about the universe?
Unlike submillimeter waves, infrared light spans a vast range of the electromagnetic spectrum from 0.04 inches (just below 1 millimeter) on the side bordering with microwaves to 0.75 micrometers on the side bordering with the visible light.
The NASA-led James Webb Space Telescope (JWST), launched on Christmas Day, 2021, thrust infrared astronomy into the spotlight with its ability to see the farthest reaches of the universe.
Infrared light, which is essentially heat, was the first non-visible wavelength discovered, completely by accident, by British astronomer William Herschel in 1800 during his experiments with the visible light spectrum. It took, however, a long time for infrared detectors to become sensitive enough to provide the breathtaking views of the cosmos that JWST is now known for.
The first crude observations of celestial objects in the infrared spectrum focused on the moon and the sun. Astronomers in the second half of the 19th century were able to measure the temperature of the sun's atmosphere as well as the various temperature zones on the moon's surface. By the turn of the century, technology progressed to the level that it was possible to detect heat from the solar system's giant planets Jupiter and Saturn, according to A brief history of infrared astronomy.
Infrared astronomy, however, didn't fully take off until the second half of the 20th century when more sophisticated detectors were developed, allowing astronomers to analyze heat sources across the Milky Way.
Related: James Webb Space Telescope images: 12 astonishing views of our universe (gallery
As the JWST has plentifully demonstrated since the release of its first images in July, 2022, infrared light is good at many things.
Thanks to its ability to penetrate through dust and gas, infrared light reveals what's going on inside of thick dust and gas clouds where stars form. Stars emerging in the middle of these clouds are not yet hot enough to emit visible light, but are warm enough to be detected by infrared sensors.
With such advanced technology as the JWST, astronomers can observe matter that is only several degrees warmer than absolute zero, the temperature of minus 459.67 degrees Fahrenheit (minus 273.15 degrees Fahrenheit), where the motion of atoms stops.
When viewing the Milky Way in infrared light, a hidden galaxy of failed stars, called brown dwarfs, emerges. Brown dwarfs are bodies that are too big to be called planets but are not quite massive enough to ignite nuclear fusion in their cores. Bodies in the farther reaches of the solar system that receive too little solar illumination also spring into view. Even the interstellar medium, the cool gas and dust dispersed between stars and galaxies, can be mapped in the infrared spectrum.
Webb was built with the aim to detect the first light that lit up the universe a few hundred million years after the Big Bang. Although this light had been emitted in the optical wavelength range, the accelerating expansion of the universe had stretched this light into the infrared range thanks to the effect known as redshift. Optical telescopes, even if they were as sensitive as Webb, could therefore no longer see this light.
But the James Webb Space Telescope sees only a small fraction of the infrared spectrum, the so-called mid and near-infrared light, which spans wavelengths from 28.5 micrometers to 0.6 micrometers where the visible spectrum begins.
NASA's recently retired flying telescope SOFIA was a specialist in the longer wavelength type of infrared light, the so-called far infrared, which reaches all the way to 612 micrometers and is best for observing the cool interstellar medium.
Famous infrared telescopes
Both, the James Webb Space Telescope and SOFIA, the current and recently retired (respectively) infrared astronomy flagships, had their predecessor.
NASA's Spitzer Space Telescope surveyed the universe in the mid-infrared and parts of the far infrared spectrum from 2003 to 2020. ESA's Herschel spacecraft complemented this work in the far-infrared spectrum between 2009 and 2013.
An earlier airborne telescope, the Kuiper Airborne Observatory, studied the infrared sky from 1974 to 1995.
What does optical light teach us about the universe?
Optical astronomy has made enormous leaps since those first early 17th-century telescopes. Enhancing the natural abilities of the human eye beyond imagination, 21st-century optical telescopes are still the backbone of astronomy research.
From giant telescopes occupying remote mountain tops and highland plateaus to orbiting super-eyes such as the iconic Hubble Space Telescope, optical observatories reveal the universe with an ever-increasing level of detail. Some, on the other hand, focus on scanning vast swaths of the sky at once to spot unexpected phenomena, such as supernova explosions of dying stars or approaching asteroids.
Optical telescopes show the universe as it would appear to human eyes. Colors in optical images correspond to the colors human eyes would see. Images from other types of telescopes, such as those imaging the universe in infrared and ultraviolet light, have to be processed by astronomers on the ground, with colors artificially assigned to different wavelengths.
To be visible in the optical wavelengths, objects need to either emit their own visible light or be illuminated by other objects. Planets, moons and asteroids in our solar system are only visible to optical telescopes (and to human eyes) because of the vicinity of our sun.
Optical light can't pass through obstacles, such as thick clouds of dust, which hide some of the most interesting areas of the universe (such as centers of galaxies where supermassive black holes devour huge amounts of material or star-forming nebulas).
Optical light is also somewhat affected by Earth's atmosphere, even though not as much as the infrared and submillimeter wavelengths. While infrared and submillimeter radiation gets mostly absorbed, optical rays get a little dispersed by the molecules in the atmosphere, which means that observed objects don't appear as sharp as they would if the atmosphere wasn't present. This atmospheric blurring limits the accuracy of observations that Earth-based optical telescopes can achieve, even though modern adaptive optics systems installed on the world's best telescopes can to a certain extent make up for this shortcoming.
Aside from complex, costly machines in space and on remote mountain tops, optical astronomy is the most accessible method of observing the sky for amateur skywatchers. Decent backyard telescopes can be purchased for a few hundred dollars and Space.com provides plenty of guides on how to pick the best one for you.
Related: Best telescopes for seeing planets in 2023
Best telescopes 2023: For stargazing galaxies, nebulas and more
Famous optical telescopes
The Hubble Space Telescope is the undisputed king of optical astronomy and the source of many images that have gained iconic status. The telescope, launched in 1990, is still going strong and still may have a decade or so of life and fabulous astronomy ahead of it.
The Very Large Telescope (VLT) operated by the European Southern Observatory (ESO) in Chile is one of the most advanced Earth-based optical telescopes. VLT consists of four main telescopes, each with a 27-foot-wide (8.2 meter) mirror, and four 5.9-foot-wide (1.8 m) auxiliary telescopes. The four main telescopes can each detect light that is four billion times fainter than what human eyes can see. The telescopes can also work together as a so-called interferometer, which increases the resolution to a level that would be achievable with a single telescope with a 426-foot-wide (130 m) mirror.
ESO is currently building the next-generation Extremely Large Telescope (ELT), also in Chile. With a single 130-foot-wide (39.3 m) mirror, ELT will be the world's largest optical telescope. Once completed, the observatory will be able to gather 100 million times more light than the human eye and provide images 16 times sharper than the Hubble Space Telescope, according to ESO.
The twin Keck Telescopes on the Hawaiian island of Maunakea are fitted with 32.8-foot-wide (10 m wide) mirrors that forced the technical teams that designed and built them in the late 1980s to develop some ingenious technical solutions. Since it wasn't possible at that time to accurately operate a single solid mirror of such a size, engineers made the Keck mirrors from 36 hexagonal segments that work together as a unit with the help of an active optics system. This segmented mirror design is quite similar to the one used for the 21-foot-wide (6.5 m) mirror of the James Webb Space Telescope.
The Large Binocular Telescope in Arizona features the world's largest non-segmented mirror, measuring 28 feet (8.4 m) in diameter.
The Gran Telescopio Canarias on the Spanish island of La Palma off the coast of western Africa, is the world's largest single-aperture optical telescope, featuring a 10.4 m wide mirror.
What does ultraviolet light teach us about the universe?
The great Hubble is also the world's main observer of ultraviolet light that emanates from sources in the universe. Ultraviolet light has shorter wavelengths and carries higher energies than visible light and points astronomers to hot, energetic processes, such as those taking place in young stars and in young star-forming galaxies. Massive stars that orbit each other in binary systems also emit ultraviolet light and so do powerful auroras on giant gaseous planets like Jupiter.
Ultraviolet light gets absorbed by the ozone layer in Earth's atmosphere, which is good for organisms living on Earth (as these wavelengths are known to cause tissue-damage and cancer). For astronomy, however, the limited ability of ultraviolet light to penetrate the atmospheres means that telescopes designed to study it need to orbit in space.
Famous ultraviolet telescopes
Apart from the Hubble Space Telescope, solar observatories such as the European Solar Orbiter or NASA's Solar Dynamics Observatory carry ultraviolet imagers to observe highly energetic processes on the sun. NASA's Jupiter explorer Juno also carries an instrument for studying ultraviolet light.
What do X-rays teach us about the universe?
Things get even more heated and energetic with X-rays. Discovered accidentally by German physicist Wilhelm Roentgen in 1895, these matter-penetrating rays are generated in vast amounts during some of the most extraordinary processes in the universe, such as when supermassive black holes or extremely massive neutron stars suck in matter from their surroundings, or during supernova explosions of dying stars.
X-rays come from the hottest places in the universe including black hole and neutron stars' accretion disks where matter spirals at extreme speeds. High-temperature plasma that fills space between galaxies in galaxy clusters also emits X-rays, and so do stars including our sun.
Astronomers recently discovered that comets can emit X-rays, Wibisono said, and that Jupiter, in addition to its ultraviolet aurora, also produces an aurora that shines in X-rays.
"X-rays are a really powerful part of the spectrum because you get fluorescence in X-rays," said Wibisono. "Rocky surfaces of moons and planets give off X-rays for fluorescence. The atmospheres around terrestrial planets also fluoresce and X-rays, the gas giants scatter solar X-rays, so they act like a mirror to the solar X-rays."
Fluorescence is the ability of a surface to absorb and subsequently emit light that originally arrived from another source.
Infamous for their potential to cause DNA mutations that may lead to cancer, X-rays get, just like ultraviolet rays, fortunately filtered out by Earth's atmosphere. X-ray astronomy could therefore only take off once humans were able to send objects to space. Astronomers knew prior to that that the sun is a powerful source of X-rays, but the first instruments capable of detecting other sources of cosmic X-rays were only launched aboard sounding rockets in the 1960s.
One of the problems with the detection of cosmic X-rays is their ability to penetrate matter. Just like they penetrate human tissue to reveal broken bones, X-rays also pass through mirrors that astronomers may want to use to concentrate them.
Building sensitive X-ray detectors therefore requires some engineering ingenuity. Scientists have to design mirrors for X-ray telescopes in a way that the energetic rays hit the reflecting surface at a shallow angle "like a stone skipping across the surface of a pond," according to NASA.
X-ray telescopes require multiple mirrors positioned at gradually increasing angles to deflect the X-rays onto a detector. Such contraptions, however, tend to be rather chunky and require large satellites to accommodate them. NASA's Chandra, for example, at 45-feet-long (13 m), is the largest satellite launched by the Space Shuttle, about a three feet (1 m) longer than Hubble.
The matter-penetrating ability of X-rays, however, also has its advantages, as these rays easily escape from dust-shrouded regions, such as galactic centers where black holes munch on the infalling matter.
Famous X-ray telescopes
NASA's Chandra X-ray observatory is the current flagship X-ray telescope. In space since 1999, Chandra travels around Earth on an elliptical orbit that takes it as far as 83,000 miles (133,000 km) away from the planet's surface where no residual atmosphere obstructs the X-ray views. During its more than two decades in orbit, Chandra has imaged jets of matter shooting from supermassive black holes in galactic centers and even traced the separation of dark matter from normal matter in the collisions of galaxies in galactic clusters.
ESA also has its X-ray space observer, the XMM-Newton space telescope, also launched in 1999.
What do gamma-rays teach us about the universe?
Gamma-rays are the highest energy type of radiation present in the universe. Just like X-rays, they come from extremely hot and energetic processes in the universe, such as supernova explosions and accreting black holes. Even more capable of penetrating matter than X-rays, gamma-rays are also produced during nuclear explosions on Earth, and, in smaller quantities, in thunderstorms and during radioactive decay. Stars such as our sun also produce occasional gamma-ray flashes in the form of solar flares.
Just like many other types of astronomy, gamma-ray astronomy came about by accident. In the 1960s, American military satellites were looking for signs of the USSR's testing of nuclear weapons, when they detected inexplicable flashes of extremely energetic gamma-rays. Lasting from fractions of seconds to several minutes, these gamma-ray bursts, as they became known, were coming regularly from all parts of the universe.
It took until the 1990s for astronomers to figure out that these bursts come from extremely powerful explosions that mark the birth of new black holes when massive stars die. The shorter types of gamma-ray bursts are produced in collisions of superdense stellar remnants called the neutron stars.
Gamma-ray bursts point astronomers to the fact that a cataclysmic event has just occurred somewhere in the universe. By measuring the intensity of the burst, astronomers can learn something about the intensity and distance of the event. However, they need to search for the source of the flash afterward, using other types of telescopes. When they manage to locate the region in the sky where the burst has come from, they can then observe the area in other parts of the electromagnetic spectrum to gain more insight into the processes involved.
Famous gamma-ray telescopes
NASA's space telescopes Fermi and Swift together with ESA's Integral are the world's current gamma-ray burst spotting workhorses. However, only Swift, which covers about 9% of the sky, has the ability to locate sources of these giant explosions.
Astronomers are therefore looking for new approaches to gamma-ray burst detection. In 2021, a team of scientists from Hungary and Slovakia launched a tiny cubesat called GRB Alpha, that has been successfully detecting gamma-ray bursts ever since. In October 2022, GRBAlpha made an accurate detection of the peak intensity of the brightest gamma-ray burst ever seen, while the event completely blinded detectors on NASA's Fermi.
The researchers envision that a fleet of such cubesats would make it possible to find sources of gamma-ray bursts across the entire sky through the so-called triangulation, the same method used to pinpoint a location on Earth with the help of GPS.
Follow Tereza Pultarova on Twitter @TerezaPultarova. Follow us on Twitter @Spacedotcom and on Facebook.
Additional resources
Astronomy Cast, produced by the Planetary Science Institute in collaboration with Universe Today has a great series on the different kinds of astronomy based on the electromagnetic spectrum. You can listen to their radio astronomy, submillimeter astronomy, infrared astronomy, optical astronomy, ultraviolet astronomy, X-ray astronomy and gamma-ray astronomy.
Bibliography
National Radio Astronomy Observatory: The History of Radio Astronomy, accessed April 2023 from: https://public.nrao.edu/radio-astronomy/the-history-of-radio-astronomy/
Walker, J. H. A brief history of infrared astronomy, Astronomy & Geophysics, Volume 41, Issue 5, October 2000, Pages 5.10–5.13: https://doi.org/10.1046/j.1468-4004.2000.41510.x
ESA: Seeing with infrared eyes: A brief history of infrared astronomy, July 2020, accessed April 2023 from: https://sci.esa.int/web/herschel/-/59550-a-brief-history-of-infrared-astronomy
Harvard University: History of X-Ray Astronomy, accessed April 2023 from: https://chandra.harvard.edu/xray_astro/history.html
ESA: History of X-ray astronomy in Europe: From Exosat to ATHENA, accessed April 2023 from:https://sci.esa.int/web/athena/-/60759-history-of-x-ray-astronomy-in-europe-from-exosat-to-athena
Pinkau, K. History of gamma-ray telescopes and astronomy, Experimental Astronomy, Volume 25, Issue 1-3, pp. 157-171, August 2009: https://ui.adsabs.harvard.edu/abs/2009ExA....25..157P/abstract
Join our Space Forums to keep talking space on the latest missions, night sky and more! And if you have a news tip, correction or comment, let us know at: community@space.com.
Get the Space.com Newsletter
Breaking space news, the latest updates on rocket launches, skywatching events and more!
Tereza is a London-based science and technology journalist, aspiring fiction writer and amateur gymnast. Originally from Prague, the Czech Republic, she spent the first seven years of her career working as a reporter, script-writer and presenter for various TV programmes of the Czech Public Service Television. She later took a career break to pursue further education and added a Master's in Science from the International Space University, France, to her Bachelor's in Journalism and Master's in Cultural Anthropology from Prague's Charles University. She worked as a reporter at the Engineering and Technology magazine, freelanced for a range of publications including Live Science, Space.com, Professional Engineering, Via Satellite and Space News and served as a maternity cover science editor at the European Space Agency.
-
rod How does BB explain the origin of the electromagnetic spectrum? For example, why should there be optical light vs. nothing to see at all?Reply
Consider some past discussions on issues like this in science.
https://forums.space.com/threads/one-of-the-greatest-damn-mysteries-of-physics-we-studied-distant-suns-in-the-most-precise-astronomical-test-of-electromagnetism-yet.59494/
https://forums.space.com/threads/how-do-we-know-the-fundamental-constants-are-constant-we-dont.59359/
https://forums.space.com/threads/what-makes-newtons-laws-work-heres-the-simple-trick.57302/ -
Pentcho Valev The underlying assumptions are that the universe is expanding and that the speed of light is constant. However the expanding-universe theory is too preposterous and will soon be abandoned. Then the only reason behind the cosmological (Hubble) redshift will turn out to be GRADUALLY DECREASING SPEED OF LIGHT. And cosmologists will have to start from the scratch.Reply -
rod "This uniformness is unseen in other wavelengths, which reveal the sky in dots and regions of varying brightness. In fact, cosmic microwave radiation is so odd that the researchers who first discovered it in the 1960s (completely by accident during experiments with echo balloons) originally thought it was produced by a telescope defect.Reply
Subsequent research, however, confirmed that the microwave hum was coming from space and that it was nothing less than the residue of radiation released by the Big Bang, the enormous explosion which created the universe some 13.8 billion years ago."
According to cosmology calculators, depending upon the input value for H0, the universe radius when the CMBR became light was about 40-41 million light years radius. In the spectrum of the CMBR, where is the H-alpha line and He showing the universe then was filled with H and He gas? The same applies to the H1 21-cm line for H gas filling the universe during the cosmic dark ages and showing zero-metal gas? -
Helio
If I understand it, the photons were highly scattered during those 40 million years until the universe cooled, due to expansion, to about 3000K (2.73 x 1100). Suddenly, the electrons became bound to the protons, forming the first atoms. Thus, all those photons were released to go flying in every direction without all that scattering. So we’re not seeing emissions from h or he, hence no emission or absorption lines.rod said:According to cosmology calculators, depending upon the input value for H0, the universe radius when the CMBR became light was about 40-41 million light years radius. In the spectrum of the CMBR, where is the H-alpha line and He showing the universe then was filled with H and He gas? The same applies to the H1 21-cm line for H gas filling the universe during the cosmic dark ages and showing zero-metal gas? -
rod Helio said:If I understand it, the photons were highly scattered during those 40 million years until the universe cooled, due to expansion, to about 3000K (2.73 x 1100). Suddenly, the electrons became bound to the protons, forming the first atoms. Thus, all those photons were released to go flying in every direction without all that scattering. So we’re not seeing emissions from h or he, hence no emission or absorption lines.
However, Helio, others do not see this as you present.
Hydrogen absorption lines in the cosmic microwave background spectrum, https://www.researchgate.net/publication/226565853_Hydrogen_absorption_lines_in_the_cosmic_microwave_background_spectrum, August 2004. "We have calculated the intensities of the subordinate hydrogen lines formed during the recombination epoch at redshifts 800≲z≲1600. We show that an allowance for the angular momentum splitting of hydrogen atomic energy levels and the dipole transition selection rules can reveal absorption features in the cosmic microwave background recombination spectrum in the submillimeter wavelength range."
Impact of inhomogeneous CMB heating of gas on the HI 21-cm signal during dark ages, https://arxiv.org/abs/1810.05908
What I see here, unlike the phases of Venus that can be clearly seen in astronomy, we do not see H and He gas filled the early universe, even during the postulated dark ages in BB cosmology. Apparently some are looking for this hard evidence. -
Helio
Thanks. I will want to look at this closer. There must be both the huge release of photons, unaffected by the new atoms, but also a significant number that did interact by hydrogen and he. We had a thread on this that noted it’s H2 that allows transparency, not H.rod said:However, Helio, others do not see this as you present.
Hydrogen absorption lines in the cosmic microwave background spectrum, https://www.researchgate.net/publication/226565853_Hydrogen_absorption_lines_in_the_cosmic_microwave_background_spectrum, August 2004. "We have calculated the intensities of the subordinate hydrogen lines formed during the recombination epoch at redshifts 800≲z≲1600. We show that an allowance for the angular momentum splitting of hydrogen atomic energy levels and the dipole transition selection rules can reveal absorption features in the cosmic microwave background recombination spectrum in the submillimeter wavelength range."
Impact of inhomogeneous CMB heating of gas on the HI 21-cm signal during dark ages, https://arxiv.org/abs/1810.05908 -
Helio
We’ve come a long way since Plato postulated the eyes emitted light to see things.Admin said:Frequencies of light invisible to the human eye reveal a vast amount of information about our universe. But it took decades for scientists to learn how
How does astronomy use the electromagnetic spectrum? : Read more
The serendipity along the way adds great color, so to speak, to the history behind understanding the spectrum. For instance, William Herschell placed a thermometer on each color of the spectrum he projected, likely using a prism. But he happen to notice that when he placed the thermometer off to the side (red side), the temperature was this highest. He discovered, as a result, the IR spectrum. -
Helio rod said:However, Helio, others do not see this as you present.
Hydrogen absorption lines in the cosmic microwave background spectrum, https://www.researchgate.net/publication/226565853_Hydrogen_absorption_lines_in_the_cosmic_microwave_background_spectrum,
In their short conclusion, they seem to confirm the spectral distribution that would exist for the Recombination event. Recombination, of course, is a major prediction of the BBT, giving us the CMBR. That they can see some Hβ and Hγ lines is interesting, and not contrary to BBT. -
rod
Helio, that did not confirm or seem to confirm H or He in the CMBR spectrum. Note what was stated:Helio said:In their short conclusion, they seem to confirm the spectral distribution that would exist for the Recombination event. Recombination, of course, is a major prediction of the BBT, giving us the CMBR. That they can see some Hβ and Hγ lines is interesting, and not contrary to BBT.
"CONCLUSIONS We have calculated the spectrum of hydrogen re-combination lines in the Wien region of the CMB radiation near its maximum. We showed that the correct analysis of the recombination kinetics can reveal the absorption features in the hydrogen recombination spectrum that correspond to the Hβ and Hγ lines. The presence of such features is an interesting fact of the conditions of almost complete thermodynamic equilibrium during the hydrogen recombination epoch. Observationally, this can increase significantly the detection probability of these spectral lines. A detailed analysis of the observing technique and the prospects for detecting these lines is beyond the scope of this study. Note only that the rapid in-crease in the number of submillimeter-wavelength telescopes and detectors and the emergence and development of new microbolometer arrays give hope that the predicted calculated distortions of the CMB radiation will be actually detected in the near future."
None have been reported as confirmed and detected and no H1 21-cm line for the cosmic dark ages. The abstract shows the redshift is in the range of 800 to 1600 compared to 1100 for the postulated redshift for the CMBR we see today. Showing the early universe whether the size of 41 million light years radius or larger during cosmic dark ages, lacks verification of H and He gas. It is a theoretical calculation that lacks verification like seeing the phases of Venus for example. BBT disciples should be very clear to the public about this I feel.