How old is the universe?
The age of the universe is determined by the expansion rate of the cosmos and the standard model of cosmology.
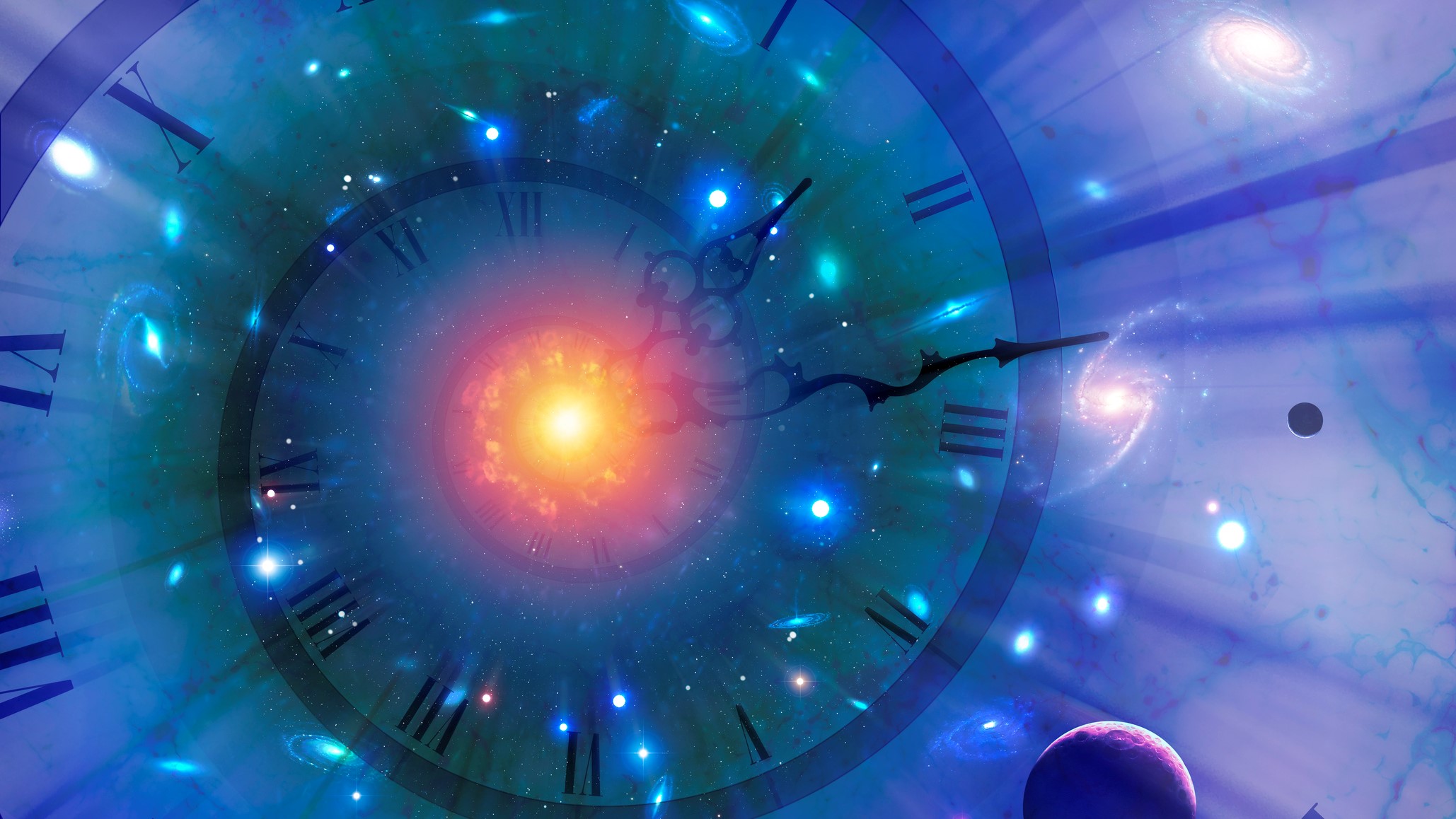
The universe is approximately 13.8 billion years old but its exact age is not yet clear. What we do know is that it's likely less than 14 billion years old.
Research from various missions has yielded slightly different estimates. Data from the European Space Agency's Planck mission gathered between 2009 to 2013 suggests that the universe is 13.82 billion years old. Another estimate, based on observations from the Atacama Cosmology Telescope in Chile, shaves a few hundred million years off the universe's age, putting it at 13.77 billion years, though astronomers at Cardiff University in the U.K. told us that the uncertainties in this measurement are still consistent with the age derived by the Planck mission.
Or, if controversial measurements of the expansion rate of the universe are correct, the cosmos could be younger. The uncertainty is not because our methods of measuring the universe's age are bad. Rather, there are still things about the universe we don't understand.
A century ago, it was assumed that the universe was eternal and static. Then, in 1924, using the world's largest telescope at that time, the 100-inch (2.5 meters) Hooker telescope at the Mount Wilson Observatory in California, Edwin Hubble discovered that almost all galaxies are moving away from us.
Related: How big is the universe?
The universe is expanding, and that has profound consequences. If the expansion of the universe is carrying galaxies away from each other, then in the past, they must have been closer together. Rewind that expansion back far enough, and every galaxy must have originated at the same point in space and time.
That point is the Big Bang, the moment our universe was created. An expanding universe cannot be eternal, but it must have a definitive start date. Without a cosmic clock to refer to, astronomers have had to embark on detective work to figure out the age of the universe, and the investigation is still ongoing.
Universe age FAQs
Could the universe be older than 14 billion years?
It is unlikely that the universe is more than 14 billion years old. For the universe to be older, we would have to throw out the standard model of cosmology — the so-called lambda-CDM model — that describes our current expanding universe. There is also other evidence that the universe is younger than 14 billion years. For example, the most distant stars and galaxies, which we see as they existed up to 13.5 billion years ago, appear young and chemically immature, which is exactly what we would expect if we are seeing them shortly after they, and the universe, formed.
How large is the observable universe?
A popular misconception is that because nothing travels through space faster than the speed of light, the observable universe should have a radius equal to the age of the universe — 13.8 billion years, give or take. However, in truth, the observable universe — the region of space in which light has had time to reach us — is 46.5 billion light-years. How can this be so? It's because, while the speed of light is the maximum velocity possible through space, space itself is not bound by this speed limit. The most distant parts of the visible universe are expanding away from us much faster than the speed of light, allowing the observable universe to inflate. A galaxy whose light set out 13.5 billion years ago, such as those seen by the James Webb Space Telescope, is now much, much farther away because space has expanded since that light left it.
How old is the universe compared with Earth?
The universe at approximately 13.8 billion years old is much older than Earth.
Earth is 4.5 billion years old. We know this thanks to a method called radiometric dating, which measures the amount of radioactive decay of isotopes in a sample to calculate how old that sample must be. The oldest rocks on Earth are 4.2 billion years old; any older rocks have been recycled through plate tectonics. However, scientists have also performed radiometric dating on lunar rocks and meteorites, and they all point to an age of 4.5 billion years for the solar system, including Earth and all of the other planets.
Are there stars older than the universe?
There have been claims that a small number of stars appear older than the universe. This would seem impossible, but if it is true, it would mean that the standard cosmology is wrong. The best-known of these stars is Methuselah, more properly known as HD 140283, located 190 light-years away. It contains very few elements heavier than the primordial hydrogen and helium from which it formed, and astronomers initially calculated its age as 16 billion years. However, rather than something being wrong with cosmology, it is more probable that our understanding of how stars age isn't quite up to snuff. Subsequent analyses have improved this modeling, and a recent scientific paper on the subject places Methuselah's age at about 12 billion years old.
Age of the universe expert Q&A
We asked Professor Geraint Lewis, of the Sydney Institute for Astronomy at the University of Sydney, Australia a few questions about the universe's age.
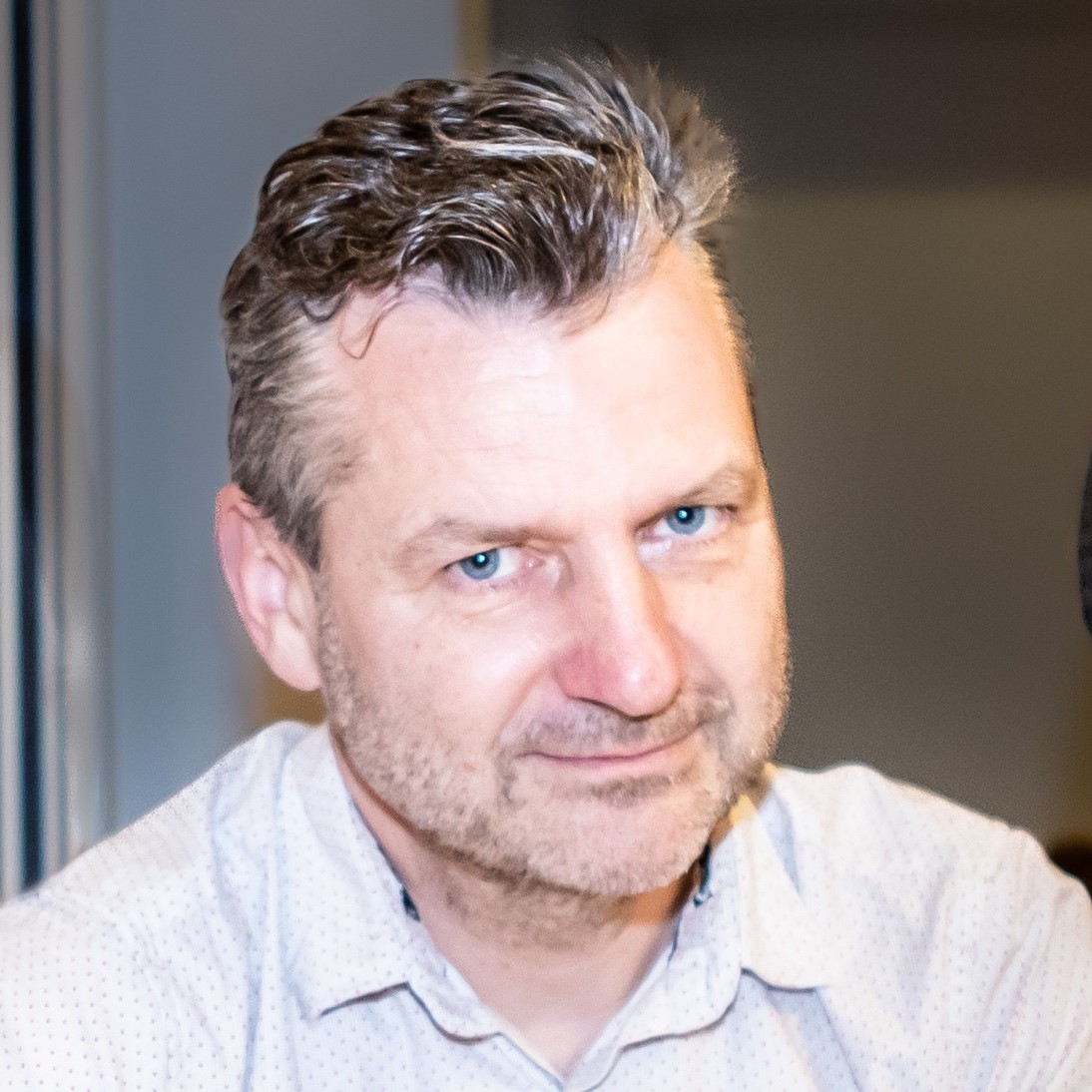
Geraint Lewis is also the author of several books, including The Cosmic Revolutionary's Handbook with fellow cosmologist Luke Barnes, which describes what any new theory designed to rival the Standard Model of cosmology needs to achieve to be taken seriously.
How do we measure the age of the universe using cosmic microwave background (CMB) radiation?
During the first few hundred-thousand years of the cosmos, the universe was a hot soup, a plasma of charged particles and radiation.
In this soup, dark matter, the dominant mass of the universe, began to be drawn together, forming the gravitational seeds of galaxies and clusters. The plasma was dragged along and sloshed about as immense waves rippled through the cosmos.
Like the ocean, there were a particular mix of waves, some long, some short.
At four hundred thousand years, the universe cooled enough for the plasma to become neutral, with electrons joining with protons to create the first hydrogen atoms. And with this, the universe became transparent, and the radiation was free to flow through the universe.
We see this radiation today as the cosmic microwave background, and the waves in the early universe are written into the radiation we receive as tiny temperature variations. From the physics of gravity and plasmas, cosmologists are able to calculate the size and mix of waves in the early universe, but how we observe these waves on Earth depends on how the universe has expanded over the last thirteen billion years, in particular the curvature of space and the rate of expansion, which is given by the Hubble Constant.
So by comparing the angular size we see to our how we understand these plasma waves to have behaves, one thing we learn is the Hubble Constant.
The CMB measurement of the Hubble Constant is 67 kilometers per second per megaparsec, but by measuring the light of supernovas, astronomers arrive at a different value, 73 kilometers per second per megaparsec. Depending on which is right, how does this affect the age of the universe?
In our cosmological theories, the Hubble Constant is a number that sets the scale of the universe, and, all other things being equal, a larger Hubble Constant generally means a younger universe.
So a universe with 73 km/s/Mpc is about 92% the age of a universe with 67 [so 12.6 billion years versus 13.8 billion years]. The real issue of the Hubble Tension is the uncertainty in each of these measurements.
Usually, these have been relatively large, so the two numbers overlapped in a statistical sense. But the current claims are that the uncertainties are now small enough that the two ages we get are not consistent, and so there is an issue *somewhere*, either mundane (like underestimating the uncertainties) or profound (something weird is happening to the universe).
There was a recent paper by Rajendra Gupta of the University of Ottawa in which he argued that observations of distant galaxies with the JWST, the existence of some stars apparently older than 13.8 billion years, and a phenomenon called 'tired light', mean the universe is actually 26.7 billion years old. Does this new theory fulfill the requirements of theories attempting to challenge the Standard Model of cosmology that you set out in The Cosmic Revolutionary's Handbook?
This new cosmological model adds a significant amount of complexity to 'solve' the problem of large galaxies in the early universe. But is this complexity really justified?
Well, firstly, I think most cosmologists feel the JWST observations are probably pointing to problems with our ideas of galaxy formation in the earliest stages of the universe rather than something amiss with the universe itself.
Secondly, the added features, like tired light, don't fit with observations that have.
Remember, if we are to take a new proposed cosmology seriously, it has to explain *all* previous observations and then some. And this new model has yet to do this. And I suspect that it won't.
How do we know the age of the universe?
The most crucial point about the expanding universe is that the more distant a galaxy is, the faster it is moving away from us. Hubble and Belgian astronomer and priest Georges Lemaître independently quantified this relationship mathematically in what has since become known as the Hubble-Lemaître law. It states that the velocity at which a galaxy is moving away from us equals the galaxy's distance multiplied by a constant of proportionality referred to as the Hubble constant (H0), which tells us the expansion rate of the universe. If we have a precise value for H0, we can rewind the history of the universe and calculate when the Big Bang took place.
So, to calculate H0 conventionally, we need to be able to measure both the distances to and the recession velocities (how fast they are receding from us) of the galaxies. We use objects called "standard candles" to measure the distances to faraway galaxies. Standard candles are objects that have a standard, easily predictable luminosity. Two good examples are Cepheid variable stars and Type Ia supernovas.
Cepheid variables, discovered by Harvard astronomer Henrietta Swan Leavitt in the early 20th century, are a type of pulsating star whose pulsations result in their brightness varying periodically. Leavitt noticed that the longer their period of variation, the brighter they were.
There is a direct relation between a Cepheid's period of variability and its intrinsic luminosity. So, when we observe a Cepheid variable in the night sky, we measure the time between peaks in its brightness to know what its maximum intrinsic luminosity should be. Then, because we know how bright it should be, we compare that brightness to how bright or faint it appears to us in the night sky to determine how far away it must be.
Type Ia supernovas work similarly. They are the explosions of white dwarfs — incredibly dense stellar remnants — and have a standardizable luminosity. Because they are far brighter than Cepheid variables, they can be used to provide distances to galaxies across a far greater range.
The velocity of a galaxy being carried away from us by cosmic expansion can then be measured from its redshift, the change in light to longer wavelengths as the light gets stretched by space's expansion. The farther away a galaxy is from us, the more its light is redshifted. And remember: The more distant the galaxy, the higher the recession velocity. Therefore, the redshift is highly dependent on the recession velocity.
Astronomers measure the distance and the recession velocity of millions of galaxies in deep surveys, and then plug the numbers into the Hubble-Lemaître law to calculate the expansion rate of the universe, H0. From that, they rewind cosmic time to find the age of the universe.
But there's a big problem nobody expected.
The Hubble tension
There's one other way to measure the age of the universe: to make measurements of the cosmic microwave background (CMB), the residual radiation of the Big Bang. For the first 380,000 years or so of its existence, the universe was so hot and so dense that photons released by the Big Bang were trapped, constantly scattering off free electrons. Only when the universe cooled enough for atomic nuclei to soak up most of the electrons, forming complete atoms, could those photons travel through space relatively unhindered.
In effect, the universe became transparent, and the radiation that was released after 380,000 years is what we see today as the CMB, which the expansion of the universe has cooled to microwave wavelengths at just 2.73 degrees above absolute zero.
By studying the temperature fluctuations in the CMB that result from the early distribution of matter and dark matter, scientists can measure both the density of matter and energy in the universe, and the value of H0. Then they can put those values into the Friedmann equation, which takes into account general relativity in the expansion of the universe. The resulting calculation gives the age of the universe.
The Planck mission, which operated between 2009 and 2013, has provided our most detailed view of the CMB yet, and has calculated H0 to be 67 kilometers per second per megaparsec — in other words, every 1 million parsecs of space (1 parsec equals 3.26 light-years, so 1 million parsecs is 3.26 million light-years) is expanding by 67 kilometers every second. From this number, Planck's scientists deduced that the universe is 13.8 billion years old.
However, by using standard candles such as Cepheid variables and Type Ia supernovas, astronomers calculate H0 to be 73 kilometers per second per megaparsec. This difference is called the "Hubble tension," and nobody knows why the expansion rate is different depending on how you measure it. If the value of 73 is correct, then the age of the universe would have to be revised down by hundreds of millions of years. That would be problematic because there would then be stars that would appear older than the universe. Assuming the tension isn't a measuring error, scientists suspect new physics may be required to explain it.
How old will the universe get?
Knowing when the Big Bang happened tells us the current age of the universe, but how old will the universe get? Will it have an end?
Cosmologists are not sure what will happen. It all depends on the nature of dark energy, the mysterious force that is causing the accelerating expansion of the universe. If that expansion continues unabated, it could bring about the end of the universe sooner than you might expect, in a "Big Rip" where the fabric of space itself is torn apart, about 22 billion years from now.
However, if dark energy weakens and the acceleration slows or even stops, the universe could have a more prolonged life. If the universe continues to expand steadily, or arrive at an equilibrium with the contractive force of gravity, the universe could possibly survive forever. After 2 trillion years, all of the galaxies beyond our gravitationally bound local supercluster will have vanished over the cosmic horizon, where the universe is expanding so fast that not even light could reach it.
By about 100 trillion years into the future, all star formation will have ended. In about 10^43 years (that's a 1 followed by 43 zeroes), protons inside atomic nuclei would begin to decay, signaling the end of matter as we know it. Finally, after about 10^100 years (known as a "googol"), even supermassive black holes would evaporate. All that would be left would be photons, neutrinos, electrons and, possibly, dark matter.
If dark energy were to somehow switch off — which is possible if it is a variable energy field called a scalar field — then gravity could regain its grip on the runaway universe and cause it to contract back down into a "Big Crunch." When this could happen, however, is unknown.
Additional resources
Learn more about ESA's Planck mission from the mission's official website. Explore the Hubble Constant in more detail with these resources from Harvard University. Learn more about the Planck constant with this informative video from The Organic Chemistry Tutor YouTube channel.
Bibliography
European Space Agency, Planck Science Highlights, https://www.esa.int/Science_Exploration/Space_Science/Planck/Planck_science_highlights
Cardiff University, Nature's Oldest Light Gives New Insight into the Age of the Universe, 20 https://www.cardiff.ac.uk/news/view/2418835-natures-oldest-light-gives-new-insight-into-the-age-of-the-universe
Rebecca Boyle, Standard Model of Cosmology Survives a Telescope's Surprising Finds, 2023, https://www.quantamagazine.org/standard-model-of-cosmology-survives-jwsts-surprising-finds-20230120/
NASA, About – Story /Edwin Hubble, 2021, https://www.nasa.gov/content/about-story-edwin-hubble
European Space Agency, Hubble diagram for Cepheids, 1999, https://esahubble.org/images/opo9919j/
International Astronomical Union, IAU Members Vote to Recommend Renaming the Hubble Law as the Hubble–Lemaître Law, 2018, https://www.iau.org/news/pressreleases/detail/iau1812/
Emily A. Margolis and Samantha Thompson, Harvard–Smithsonian Center for Astrophysics, Remembering Astronomer Henrietta Swan-Leavitt, 2021, https://www.cfa.harvard.edu/news/remembering-astronomer-henrietta-swan-leavitt
Australia Telescope National Facility, Cepheid Variable Stars and Distance Determination, https://www.atnf.csiro.au/outreach/education/senior/astrophysics/variable_cepheids.html
Britannica, Type I supernovae, https://www.britannica.com/science/supernova/Type-I-supernovae#ref1030043
Adam G Riess et al, A Comprehensive Measurement of the Local Value of the Hubble Constant with 1 km/s/Mpc Uncertainty from the Hubble Space Telescope and the SH0ES Team, Astrophysical Journal Letters, 2022,
https://iopscience.iop.org/article/10.3847/2041-8213/ac5c5b
Vanderbilt University, New model of cosmic stickiness favors ‘Big Rip' demise of universe, 2015
Lawrence M. Krauss and Glenn D. Starkman, The Astrophysical Journal, 2000, https://doi.org/10.1086%2F308434
Fred C. Adams and Gregory Laughlin, A Dying Universe: the Long-Term Fate and Evolution of Astrophysical Objects, Reviews of Modern Physics, 1997, https://doi.org/10.1103%2FRevModPhys.69.337
Join our Space Forums to keep talking space on the latest missions, night sky and more! And if you have a news tip, correction or comment, let us know at: community@space.com.
Get the Space.com Newsletter
Breaking space news, the latest updates on rocket launches, skywatching events and more!
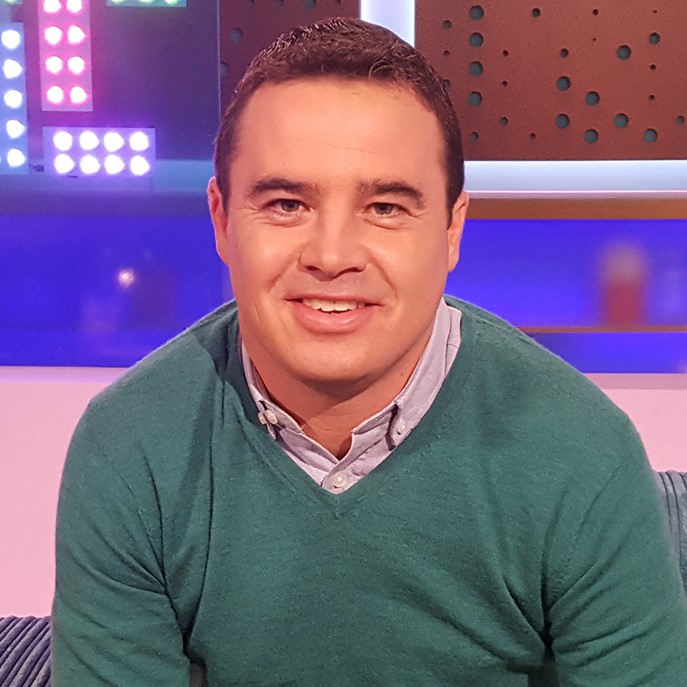
Keith Cooper is a freelance science journalist and editor in the United Kingdom, and has a degree in physics and astrophysics from the University of Manchester. He's the author of "The Contact Paradox: Challenging Our Assumptions in the Search for Extraterrestrial Intelligence" (Bloomsbury Sigma, 2020) and has written articles on astronomy, space, physics and astrobiology for a multitude of magazines and websites.