Gamma rays: Everything you need to know about these powerful packets of energy
Gamma rays can only be detected by sensors made of dense metals and takes over six feet (1.8 meters) of concrete to block.
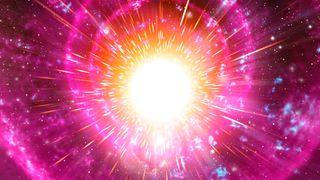
Gamma rays are high-energy photons produced by some of the most violent events in the universe.
Photons of light are massless particles that are essentially packets of energy. Because of a quantum-mechanical phenomenon known as wave-particle duality, particles can behave like waves, and photons are no different. Photons have wavelengths, and the amplitude of their wavelength determines where they sit on the electromagnetic spectrum. Radio and microwave photons sit at the lower energy, longer wavelength end of the spectrum, while in the shorter wavelength, higher-energy regime are photons of ultraviolet, X-rays and the most energetic of them all with the shortest wavelengths: gamma rays.
Gamma rays have wavelengths shorter than 10^-11 meters and frequencies above 30 x 10^18 hertz. The European Space Agency describes how gamma-ray photons have energies in excess of 100,000 electronvolts (eV). We can compare this to X-rays, which NASA describes as having energies between 100 eV and 100,000 eV, and optical photons that we can see with our eyes, which are about 1 eV.
Related: What is the cosmic microwave background?
On Earth, gamma rays are produced by radioactive decay, nuclear weapons and lightning, while in space they are produced by violent, high-energy sources such as solar flares, quasars, black holes tearing stars apart, black-hole accretion disks, exploding stars and the strong gravitational environments of neutron stars.
How were gamma rays discovered?
At the turn of the twentieth century, two forms of radiation emitted by decaying atoms were known, namely alpha particles (which are helium nuclei) and beta particles (which are electrons and positrons).
However, when the French chemist Paul Villard began experimenting with the radioactive element radium, which had been discovered two years prior by Marie and Pierre Curie, he noticed that the ionizing radiation produced by the decay of radium packed a harder punch than either alpha or beta particles.
This radiation received its name — gamma-rays — simply because gamma is the third letter in the Greek alphabet after alpha and beta. Unbeknownst to Villard and his cohorts in the early 1900s, the key difference between gamma rays and alpha/beta particles is that gamma rays are a form of light, whereas alpha and beta particles are made of matter.
How to stop gamma rays
To block gamma rays requires a dense material, and the thickness of that material depends on the substance. To reduce the strength of incoming gamma rays by a billion, you need 13.8 feet (4.2 meters) of water, 6.6 feet (2 m) of concrete or 1.3 feet (0.39 m) of lead, according to the radiation protection solution website StemRad.
This poses a problem for gamma-ray telescopes, such as NASA's Fermi Space Telescope. Ordinary telescopes like the Hubble Space Telescope use mirrors and lenses to collect and focus light, but gamma rays will simply pass straight through an ordinary telescope. Instead, gamma-ray telescopes have to employ other means.
On the Fermi Space Telescope, a gamma-ray photon will pass through a device called the Anti-coincidence Detector, which blocks cosmic rays that might give a false signal, according to NASA . The gamma-ray is then absorbed by one of 16 sheets of tungsten, a material that is dense enough to stop gamma rays.
By interacting with the tungsten, the gamma-ray is converted into an electron and a positron (the antimatter or antiparticle counterpart of an electron), the paths of which are read by a tracker, which is a module of silicon strips interweaved by tungsten foil that can determine the direction that the gamma-ray came from in space, based on the trajectory of the electron and the positron.
Finally, the electron and then positron have their energies measured by a calorimeter — a device that measures the energy of a particle by absorbing it — made from cesium iodide, and therefore the energy of the gamma-ray can be determined.
Are gamma rays dangerous?
Because of their high energy, gamma rays are ionizing, meaning they can dislodge electrons from atoms, ultimately damaging living cells and causing a hazard to health. However, as with all radiation, it depends upon the dose that you receive.
In small doses, very carefully targeted to limit exposure, they can be used safely as a medical diagnostic tool, or even to kill cancerous cells (ironic since exposure to radiation including gamma rays can cause cancer). In particular, one tool used by doctors is the 'Gamma Knife', which is an ultra-precise form of surgery in which a beam of gamma rays cuts away diseased brain cells and can even penetrate deep into the brain without damaging the exterior lobes.
Gamma-ray astronomy
Given their ionizing power, it's fortunate that Earth's atmosphere is able to block gamma rays from space. For astronomers, however, that's unfortunate, because it means that to conduct gamma-ray astronomy observatories have to either be built on mountaintops where the atmosphere is thinner or sent into space.
The first gamma-ray space telescope was launched in 1961 on the NASA Explorer 11 satellite, but things didn't really begin to kick off until the late 1960s and early 1970s with a major finding, and it wasn't even an astronomical telescope that made the discovery.
Over the years there have been many observatories, both on the ground and in space, that have been designed to observe cosmic gamma-ray radiations. In 1990, NASA launched the Compton Gamma-Ray Observatory as the gamma-ray counterpart to the Hubble Space Telescope. The Compton Gamma-Ray Observatory explored the cosmos from 1991 until 2000. The aforementioned BeppoSAX was a joint Italian–Dutch mission that operated between 1996 and 2003, while NASA launched HETE-2 (the High-Energy Transient Explorer; HETE-1 had previously failed in orbit) that tracked down many GRBs between 2000 and 2008.
Currently, as of the end of 2022, several satellites, observatories and telescopes continue to conduct gamma-ray astronomy both on Earth and in space. NASA's Swift satellite, launched in 2004, combines both X-ray and gamma-ray observations, as does Italy's AGILE satellite launched in 2007. In 2002, the European Space Agency launched INTEGRAL, the International Gamma-Ray Astrophysics Laboratory.. The current most sophisticated gamma-ray space telescope is Fermi, which NASA launched in 2008.
Meanwhile, on the ground, there are several gamma-ray observatories including VERITAS (Very Energetic Radiation Imaging Telescope Array System) at the Fred Lawrence Whipple Observatory in Arizona and HESS (High Energy Stereoscopic System) in Namibia.
Gamma-ray bursts
In 1963, the Soviet Union, the United Kingdom and the United States signed a nuclear test ban treaty that prohibited the world's superpowers from testing any nuclear devices in the atmosphere or in space. However, the U.S. was suspicious that the Soviet Union wouldn't adhere to the treaty, so they launched the Vela series of satellites to watch for any pulses of gamma-ray radiation that could be coming from secretive nuclear detonations. As it happened, gamma rays were detected, but from space: random blasts of powerful gamma-ray energy that seemed to be coming from all around the Earth. But how far away were these gamma-ray bursts?
Related: Most powerful gamma-ray burst ever seen could help reveal how black holes are born
If these gamma-ray bursts, which are abbreviated to GRBs for short, were coming from our galaxy, then astronomers would detect them mostly in the plane of the Milky Way. Instead, they were spread all over the sky, it could mean only one of two things. Either they were very close, within our solar system, or they were very far away, beyond our galaxy. A special debate was even convened in 1995, echoing a similar 'Great Debate' in 1920 between Harlow Shapley and Heber D. Curtis that discussed the size of our galaxy based on the distribution of globular clusters.
In the 1995 debate, chaired by Martin Rees, astronomer Bohdan Paczynski of Princeton University argued that GRBs came from very far away, while Donald Lamb of the University of Chicago reasoned that GRBs must be from close by because the energy required for them to be billions of light-years away would contravene the laws of physics.
Just two years later astronomers had their answer when the BeppoSAX satellite detected a gamma-ray burst that the William Herschel Telescope in the Canary Islands was able to quickly follow up on, in the process detecting the faint afterglow of whatever explosion had created the GRB. Measuring the redshift of the afterglow's light revealed it to have come from six billion light-years away. Bohdan Paczynski was right!
There are two main types of GRB. One type is called short GRBs which last just fractions of a second, while the other kind is known as the long GRBs, and can last many seconds up to an hour. Short GRBS are emitted during the merger of two neutron stars, while long GRBs are the death cries of rare, massive stars.
Physicists Andrew MacFadyen and Stan Woosley of the University of California, Santa Cruz, developed a model to explain how stars could explode and produce long GRBs without breaking the laws of physics. When a massive star with 50–100 times the mass of the sun reaches the end of its life, the star begins to collapse in on its core, and if the star is rotating fast enough, the energy within the collapsing layers rebounds off the core and is blasted out in two jets that move at almost the speed of light and blow the star apart. Charged particles within these jets spiral around powerful magnetic fields and produce something called synchrotron radiation, in the form of the gamma rays that we observe. Because the gamma rays are only released in the direction of the jets, and not in all directions at once, the total energy released does not contravene the laws of physics.
Additional resources
Learn more about ionizing radiation with the United States Environment Protection Agency (EPA), and the American Cancer Society. Explore gamma rays in more detail in a tour of the electromagnetic spectrum with NASA Science.
Follow Keith Cooper on Twitter @21stCenturySETI. Follow us on Twitter @Spacedotcom and on Facebook.
Bibliography
Flash! The Hunt for the Biggest Explosions in the Universe by Govert Schilling (Cambridge University Press, 2002)
The Biggest Bangs: The Mystery of Gamma-Ray Bursts, The Most Violent Explosions in the Universe by Jonathan Katz (Oxford University Press, 2002)
Join our Space Forums to keep talking space on the latest missions, night sky and more! And if you have a news tip, correction or comment, let us know at: community@space.com.
Get the Space.com Newsletter
Breaking space news, the latest updates on rocket launches, skywatching events and more!
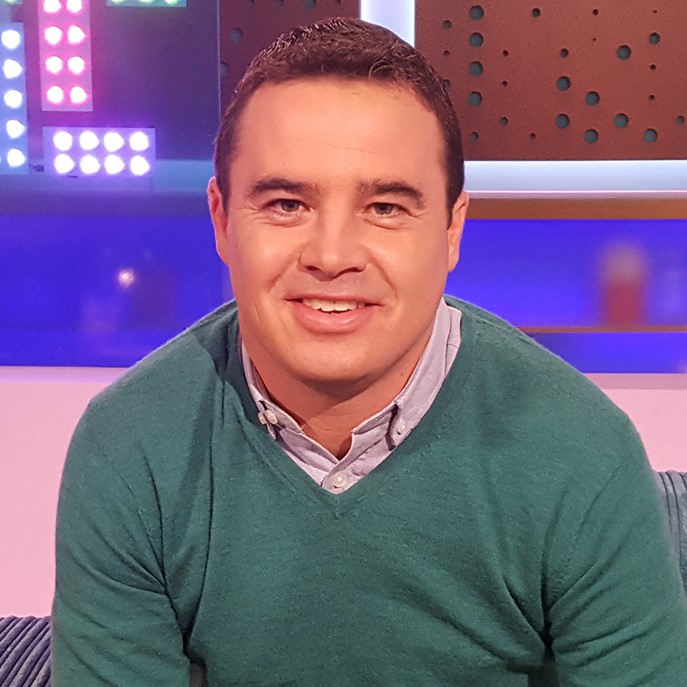
Keith Cooper is a freelance science journalist and editor in the United Kingdom, and has a degree in physics and astrophysics from the University of Manchester. He's the author of "The Contact Paradox: Challenging Our Assumptions in the Search for Extraterrestrial Intelligence" (Bloomsbury Sigma, 2020) and has written articles on astronomy, space, physics and astrobiology for a multitude of magazines and websites.
-
billslugg The 1859 Carrington event was caused by a stream of charged particles from a solar flare. Any gamma rays emitted would have been blocked by the Earth's atmosphere.Reply