The Cosmological Conundrum of the Expansion Rate of the Universe
Different measurements don't add up.
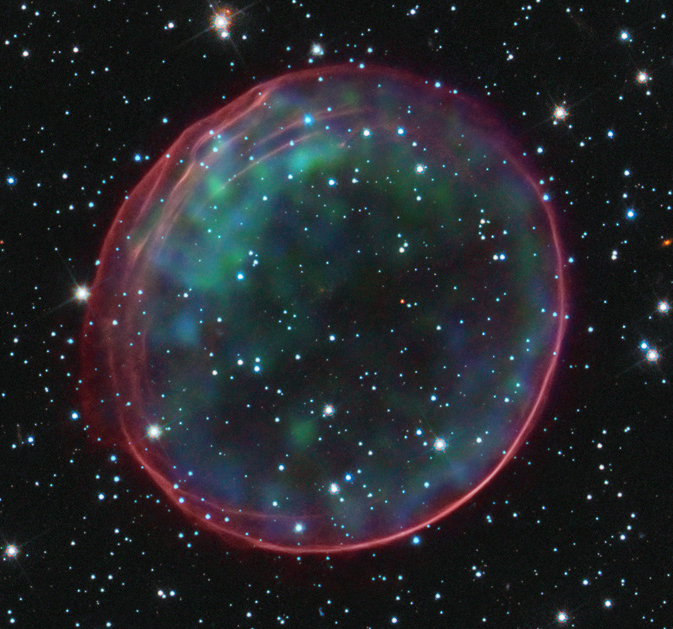
Paul M. Sutter is an astrophysicist at The Ohio State University, host of Ask a Spaceman and Space Radio, and author of "Your Place in the Universe." Sutter contributed this article to Space.com's Expert Voices: Op-Ed & Insights.
In the 1920s, astronomer Edwin Hubble discovered something absolute groundbreaking and unprecedented in the history of really all of humanity. He found that our universe is alive. It's dynamic, it's changing, it's evolving with time.
Our universe was different in the past and will be different in the future. And that difference is due to expansion. Over time, galaxies on average get farther away from us and farther away from each other. We live in an expanding universe. But exactly how fast is it expanding today? Astronomers are struggling with this answer because different techniques are giving different results, and the resolution to this problem may come in the form of brand-new physics. Or maybe we're just getting something wrong. Hard to tell.
Related: The Universe: Big Bang to Now in 10 Easy Steps
Theoretical glue
We understand the expansion of the universe through Einstein's theory of general relativity. This is also how we understand all gravitational interactions in said universe. General relativity connects the amount and kinds of stuff you have in space with distortions of that space, giving rise to bends and folds and features. Then that geometry of space-time tells all the stuff how to move. Voila: gravity.
The universe is by definition made of stuff, and therefore is subject to general relativity. The amount and kinds of stuff at grand scales tell space-time how to bend at those same grand scales, and that bending of space-time tells all the matter how to move. So the ingredients of the universe affect the expansion rate. And in different points in cosmic history, different components have been in charge; the universe is made of many things like normal matter, radiation, dark matter, and dark energy. Over the course of the past 13.8 billion years, some of these have been more dominant than others, affecting the expansion rate at that particular time.
The equations of general relativity give us the glue to go from the ingredients at one moment of cosmic time to the expansion rate at that time or vice versa, to go from the expansion rate at that time to the ingredients. This is the magic of equations: they go in both directions of the equal sign. This means that cosmologists can either go out to try to measure the expansion rate directly, or try to measure the ingredients directly and calculate the expansion rate.
Get the Space.com Newsletter
Breaking space news, the latest updates on rocket launches, skywatching events and more!
Related: In Photos: Universe's Expansion Revealed by Quasars & Cosmic Lenses
A baby picture
One of our best tools for measuring the ingredients of the universe is the cosmic microwave background. This is the leftover radiation from when the universe was just 380,000 years old. It's a veritable baby portrait of the entire cosmos. That light was released long ago, and it's been sailing through the void of space until it hit our detectors like Europe's Planck satellite, which very accurately measured the cosmic microwave background a few years ago.
From that portrait we are able to accurately measure the ingredients in the universe. How much normal matter, how much dark matter, how much neutrinos, how much radiation and so on. With those ingredients in hand, we can plug them into general relativity to tell us the behavior of the universe. Then we can run the clock forward from the emission of the cosmic microwave background through all 13.8 billion years of history to the present day and predict what the expansion rate is right now.
The upside of the cosmic microwave background is that it's an extremely clean dataset with a wealth of observations made on it. It's a treasure trove sitting right there in the sky. But while it gives us a very accurate picture of the universe when it was a baby, we do have to use models to try to understand what the universe is like as a grown-up. Still, this is a rather uncontroversial approach, so the number that it gives for the expansion rate today ought to be very accurate.
Out with a bang
And it is very accurate, but it also disagrees with other measurements. Which is kind of a problem.
We have ways of directly measuring the expansion rate of the universe around us, which means we have ways of directly measuring the expansion rate right now today. We measure this through type 1A supernovae, a certain kind of cataclysmic explosion that has a few very compelling and useful properties.
For one, type 1A supernovae are very bright, letting us see them from billions of light-years away. For another, they're not too uncommon, so we can see them popping off quite frequently. Third, we have ways of estimating their true brightness (as in the brightness that you'll get if you were standing right in front of one), and we can compare that to the brightness that we see on our sky and use that to calculate a distance. Then, by looking at the redshift of the galaxies that host the supernovae, we can build up a relationship between distance and speed away from us. That is the expansion rate (also known as the Hubble constant or Hubble rate, in honor of the guy who first tried to take a crack at it).
The supernova measurements have the advantage of skipping all the general relativity theory work and just directly measuring the thing we're interested in. And while type 1A supernovae are very good at providing measurements, they're not perfect. They're not exactly as standard and reliable as we would like, meaning it takes a lot of sweating and working to do our best to accurately calculate their distance.
Still, astronomers are very clever and resourceful, so they're able to work all this out. But the number they get is higher than the one predicted from the cosmic microwave background. It's not much different — just a few percent off — but we're so good at measuring these values nowadays and the uncertainties are so small that a disagreement is apparent and unignorable.
Great. Now what?
Related: The Universe Is Expanding So Fast We Might Need New Physics to Explain It
Rising tensions
Cosmologists have been in this sticky spot before. Back in the '80s and '90s, some groups of astronomers predicted a Hubble expansion over twice as big as what other predictions were. The resolution came through simply more data. As we learned more about the universe, the group on the high end started to bring their number down and the group on the low end started to bring their number up. Eventually they met, and graceful harmony ensued.
Are we in the same situation today? Will more data slowly and achingly notch these numbers around until the disagreement vanishes? Maybe. Or maybe not.
It's certainly the case that neither group of astronomers will admit to any egregious errors in their methods or analysis. So until more data come in, the only remaining thing to examine is the glue, our theory that connects the early universe observations of the cosmic microwave background to the modern-day observations of the expansion rate.
General relativity itself is pretty unassailable these days, especially with the test provided by the recent kilonova of a neutron star merger which put the kibosh on almost every attempt to go past Einstein's magnum opus. That means we have to look at the ingredients in the recipe, not the recipe itself, for a culprit, and one of the key unknowns here is the nature of dark energy.
Back when the cosmic microwave background was released, dark energy was a non-player. It simply didn't have enough presence to affect cosmic history, but today it's the major power player of the cosmos, dominating our universe and accelerating our expansion. If we assume that dark energy has always been the same through time, then we get the discrepancy that we observe. If we allow for some variation in the nature of dark energy through time, then we can explain the the differences in measurements. But when we try that, the models become much more uncertain, and we don't have enough observational oomph yet to make a firm call one way or another.
Of course we have no idea what dark energy is. And we have no idea if it really is changing with time. Whatever the solution is, in this case we definitely have to go past the bounds of what we currently understand about fundamental physics. Could this tension in cosmological observations be the first cracks letting the light in to a new understanding? Or will we have to fumble around for a few more decades, only to realize it was just another mundane flaw in our analysis? Only time will tell.
Also, a lot more data will help.
- Gravitational Waves Could Solve Hubble Constant Conundrum
- Exotic 'Early Dark Energy' Could Be the Missing Link of Universe's Expansion
- Universe's Expansion Rate Is Different Depending on Where You Look
Learn more by listening to the episode "How do we measure the expansion of the universe?" on the Ask A Spaceman podcast, available on iTunes and on the Web at http://www.askaspaceman.com. Thanks to Martin N., Gary S., Vernon S., Jim K., Tim R., @jamosmith, Mike N., and Cody for the questions that led to this piece! Ask your own question on Twitter using #AskASpaceman or by following Paul @PaulMattSutter and facebook.com/PaulMattSutter.
Follow us on Twitter @Spacedotcom or Facebook.
Join our Space Forums to keep talking space on the latest missions, night sky and more! And if you have a news tip, correction or comment, let us know at: community@space.com.
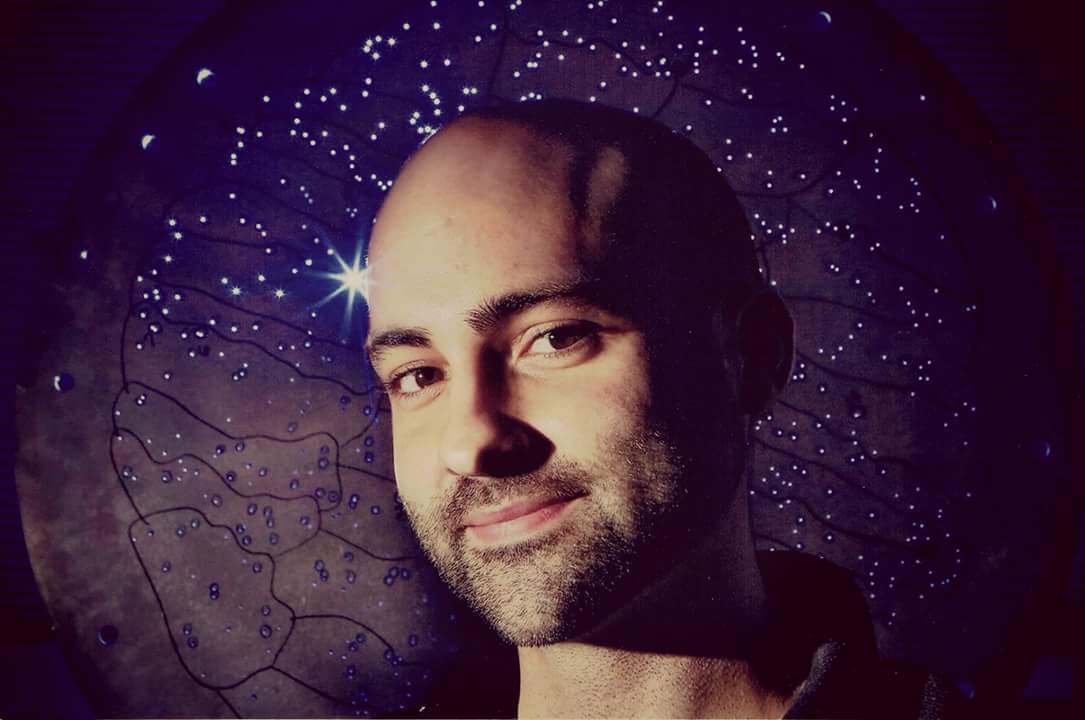
Paul M. Sutter is a cosmologist at Johns Hopkins University, host of Ask a Spaceman, and author of How to Die in Space.