Somewhere in the multiverse, dark energy is helping stars and life form
The findings call into question just how finely tuned our universe really is.
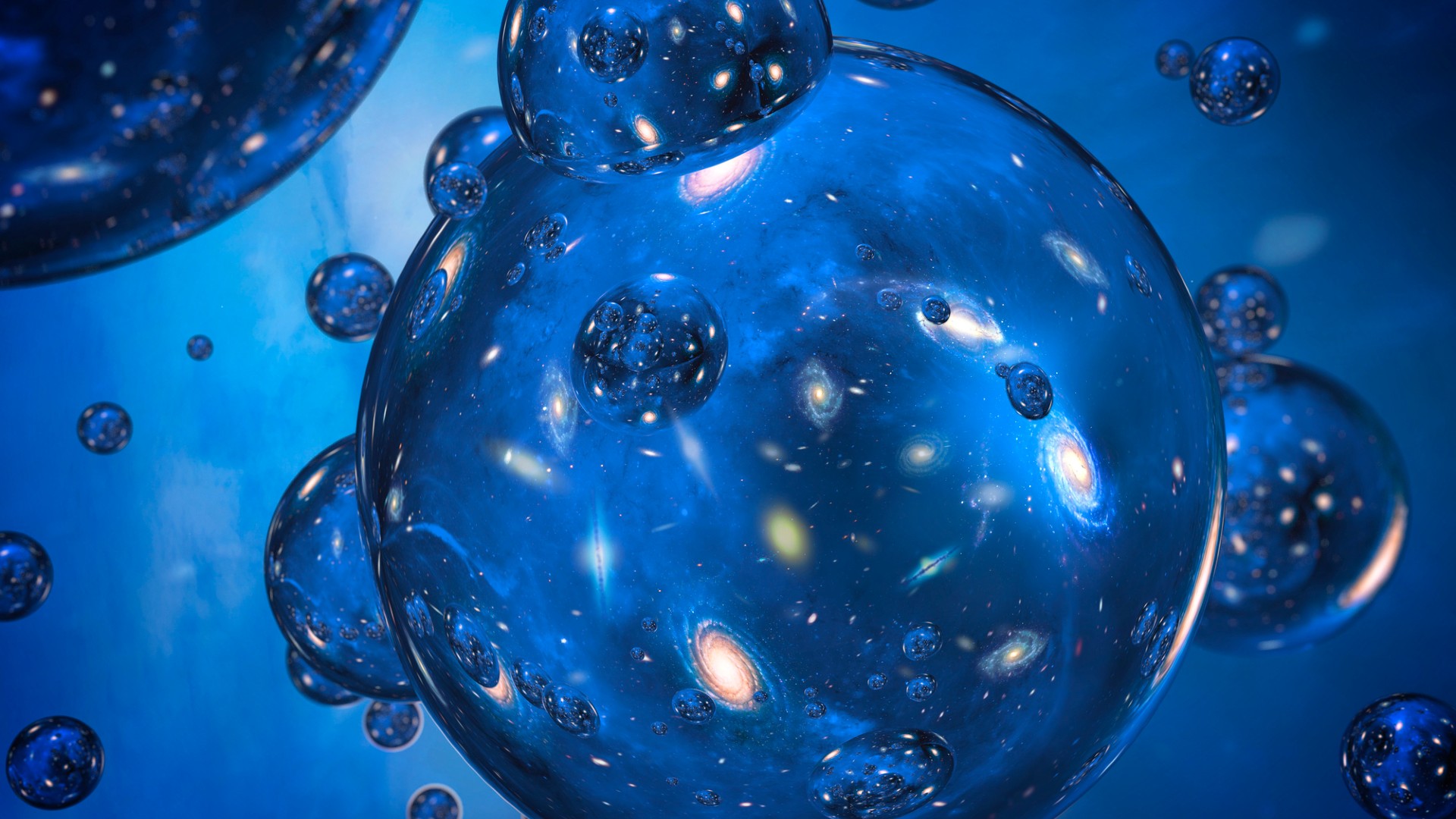
Somewhere in the multiverse, there could be universes more predisposed to forming stars, and possibly life, than our own universe —- and a new study has shown that it's all thanks to the most unlikely of suspects: dark energy.
A team of scientists has explored how the strength of dark energy directly affects how easily stars form, and it turns out that the strength of dark energy in our universe doesn't lead to the most efficient star factories. In fact, the researchers say that if you select a random observer from anywhere in the multiverse, chances are they'd come from a universe where the strength of dark energy is much greater than it is in our universe.
"That was quite surprising to me!" Daniele Sorini of Durham University, who led the study, told Space.com.
Dark energy is the mysterious force that is accelerating the expansion of the cosmos. The most popular model for dark energy suggests it is the cosmological constant, which describes the intrinsic energy of empty space that is powering the acceleration of the expanding universe. We say that it is "constant" because we think the strength of dark energy has remained the same throughout history.
Suppose, though, that dark energy had a different strength, making the universe's expansion accelerate either faster or slower. Sorini, along with John Peacock of the University of Edinburgh and Lucas Lombriser of the University of Geneva, have modeled how changing dark energy in this way would affect star formation, and because stars are essential for life, how it would affect the habitability of the universe.
To the multiverse!
The crew turned to the multiverse.
The multiverse concept assumes that our universe exists as part of an ensemble of perhaps infinite universes, each with its own distinct characteristics, including possibly different strengths for dark energy.
Breaking space news, the latest updates on rocket launches, skywatching events and more!
For life to exist in a universe — life as we know it, at least — that universe needs stars to provide heat and energy to orbiting planets for such life to live on. Sorini, Peacock and Lombriser found in their calculations that the universes most efficient at forming stars have a strength of dark energy —- referred to as the density of dark energy, or how much energy each little piece of space contains — that is one-tenth of the value of dark energy in our universe.
This means that in those more efficient universes, the expansion of space is still accelerating, but only one-tenth as fast. All of the matter in those universes would therefore be much closer together than in our universe.
A universe like this is, the team says, would turn 27% of its gas into stars over cosmic history. Our universe, on the other hand, is expected to convert about 23% of its gas into stars.
But there is a twist in the tale: Suppose you select a random observer from anywhere in the multiverse. An observer (or at least life as we know it) can only exist in a universe that forms stars. If you asked that random observer to describe the strength of dark energy in their corner of the multiverse, chances are that they'll live in a universe where dark energy is stronger than in our universe.
Wait, what?
This sounds like a contradiction. If universes with weak dark energy are the most adept at forming stars — recall that the most efficient universes would have one-tenth the dark energy strength as ours — how can we expect to find most observers in universes with stronger dark energy?
It's all down to statistics, but Sorini has an analogy to help.
"Suppose that you have got many boxes, marked from 1 to 100, and that you are putting marbles into those boxes," he said.
Imagine that you put the most marbles, say 100 of them, into box number 2. Fewer marbles, maybe 10 a-piece, go into the other boxes. In Sorini's analogy, box 2 with the most marbles corresponds to the peak of star-formation efficiency with a dark-energy density one-tenth of our universe's. We might then find our universe as corresponding to box 4 or 5, for example, containing fewer marbles.
However, even though all the boxes numbered 3 to 100 each individually contain fewer marbles than box 2, add them all together and there are more marbles in boxes 3 to 100 combined than there are in just box 2. So, if we were to choose a marble at random, the likelihood is that it will come from a box numbered higher than 2.
"It's a similar thing with the multiverse," said Sorini. In other words, the most efficient star-forming universes have a low value for dark energy, but there are many more universes with much greater values that, while not quite as efficient, still produce stars and result in a habitable universe.
This is only the case up to a point though; dark energy can also theoretically become so strong that it doesn't give any stars a chance to form, or rips apart the universe shortly after some stars do form. Such universes might be represented by boxes 101 and above in Sorini's analogy, each containing no marbles at all.
Lower efficiency, higher quality
Star formation occurs when huge clouds of gas collapse; galaxies form inside massive haloes of this star-forming gas, and the sizes of these haloes are dictated by the interplay between the physics of star formation and the growth of large-scale structures in the universe. Large-scale structures grow more quickly when dark energy is weaker, and more slowly when dark energy is stronger. This is because it's easier for strong dark energy to pull everything apart faster.
However, "the interesting result is that when you've got higher values of dark energy, individual haloes can host galaxies that are more efficient at forming stars," said Sorini.
The reason for this is that, as dark energy increases in strength, the radius of a given halo becomes smaller and more compact because its radius is proportional to both the halo's mass and the Hubble constant, which quantitatively describes the expansion rate of the universe. Because the haloes are more compact, the density of star-forming gas within them is greater — and the denser the gas, the faster the gas cools, so that halo then favors enhanced star formation that requires cold temperatures (below ten degrees above absolute zero) to permit gravitational collapse of the gas into stars. However, because dark energy is working against the formation of large-scale structures, fewer galaxies form in such a universe — therefore, there are fewer stars overall there.
Stars enrich the chemistry of the universe when they expire, releasing the heavy elements formed via nuclear fusion reactions deep inside them. The biochemistry of life on Earth is based on five elements — hydrogen, carbon, nitrogen, oxygen and phosphorus — which build the chemical building blocks that form the nucleotides that assemble into DNA. With the exception of hydrogen, which was produced in the Big Bang, all of these elements are produced by stars. These and other, heavier elements are also the materials used to build planets, which need to orbit stars to receive the light and heat necessary for life as we know it. The more stars form, the more of these elements there are, and the more planets there will be that could support life.
Against the anthropic principle?
The strength of dark energy in our universe has been a source of consternation for scientists ever since the mysterious accelerative force was discovered in 1998. Most theories predict that the cosmological constant in our universe should have a large value, but in reality the strength of dark energy that we observe in our universe is 10^120 times smaller than predicted. That's a big difference — are our best theories really that badly wrong?
We should feel fortunate that dark energy is so weak in our universe. If it were as strong as our theories say it should be, it would have led to a runaway universe that would potentially have torn the fabric of space-time apart in a "Big Rip" long ago, and no life would be able to exist. We'd be in box 101.
But dark energy isn't the only parameter in the universe that seems friendly to support life. The speed of light, the charge of an electron, the mass of a proton, the strength of gravity and the strong force, among others, all seem finely balanced. Were their values even slightly different, the universe would be a changed and more inhospitable place, with stars and life unable to form and develop. What’s more, we don't know why they have the values that we measure. What we do know is that if they didn't have those values, then we wouldn’t be here to measure them.
This paradox is called the anthropic principle, which describes a selection effect in which we measure these values as being so finely tuned for life because we could not exist in a universe where they are not finely tuned — they are a necessity for our existence.
One proposed solution is that our universe is but one universe in a multiverse, which consists of a possibly infinite ensemble of parallel universes which each have fundamental constants with different values. We would be bound to inhabit one of these universes that is friendly to life rather than one that isn't.
It had been assumed that the strength of dark energy was another of the anthropic principle's finely tuned parameters, especially with that puzzling huge discrepancy between theory and observation. But Sorini, Peacock and Lombriser's findings don't seem to quite align with anthropic reasoning because they have shown that other universes could have even more favorable dark-energy strengths.
"I think the key take-home message is to be careful when you apply anthropic reasoning, because the question is actually more subtle than it might look on the surface," said Sorini. "Our findings suggest that you should not use this argument as a get-out-of-jail-free card without more careful reasoning on the assumptions that go behind it."
However, there are some caveats to their findings. For one thing, they assume that dark energy is the cosmological constant, and not a scalar field that can change strength over time. They also assume a certain astrophysical model of star formation; a different model might yield different results. Or perhaps, if the multiverse is real, certain values of dark energy might for some reason be more prevalent than others, meaning that we would need to consider a more complex multiverse in which, in Sorini’s box analogy, a larger number of boxes might have 100 marbles as opposed to 10 marbles.
Isolated islands in an empty sea
While other possible universes might offer more chances for life, it's impossible to say whether they are more inhabited than our own. There are, after all, many more factors involved in the emergence of life than just the efficiency with which a universe forms stars. Suppose though one of these universes with a different dark-energy strength than our universe did have life — what would its inhabitants see? Because dark energy is causing the expansion of the universe to accelerate over time, universes where dark energy is weaker would see its galaxies and galaxy clusters closer together and universes where dark energy is stronger would have experienced much more expansion than our own, transporting galaxies much farther apart from one another.
"The structures that form would be isolated islands in an empty sea," said Sorini. "It could be that we wouldn't even see other galaxies, because everything would be disconnected from us. We would be under the illusion that our galaxy is the only galaxy in the entire universe."
So, while we might be somewhat lucky to exist in our less-optimized universe, having a sky full of galaxies seems much more preferable to being an island in an ocean of nothing.
The research was published on Nov. 13 in the journal Monthly Notices of the Royal Astronomical Society.
Join our Space Forums to keep talking space on the latest missions, night sky and more! And if you have a news tip, correction or comment, let us know at: community@space.com.
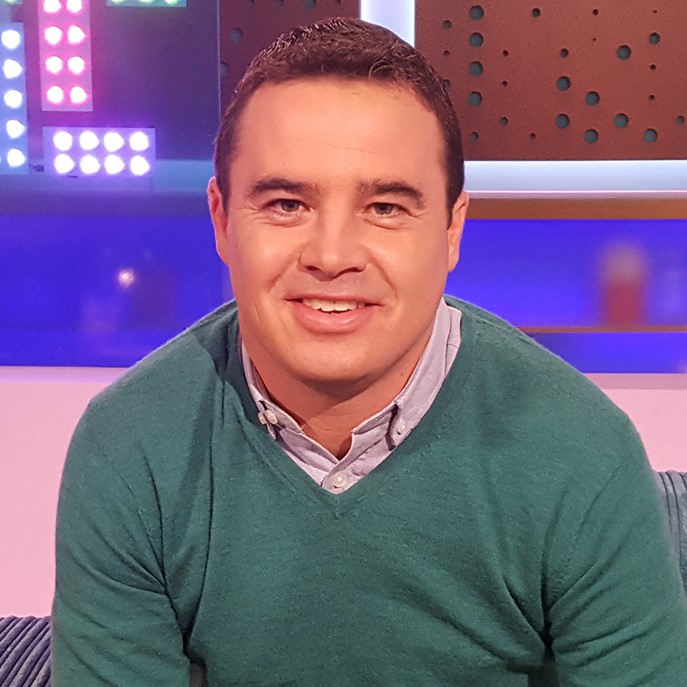
Keith Cooper is a freelance science journalist and editor in the United Kingdom, and has a degree in physics and astrophysics from the University of Manchester. He's the author of "The Contact Paradox: Challenging Our Assumptions in the Search for Extraterrestrial Intelligence" (Bloomsbury Sigma, 2020) and has written articles on astronomy, space, physics and astrobiology for a multitude of magazines and websites.