What is a black hole event horizon (and what happens there)?
The interior secrets of black holes are guarded by a one-way light-trapping boundary called the event horizon.
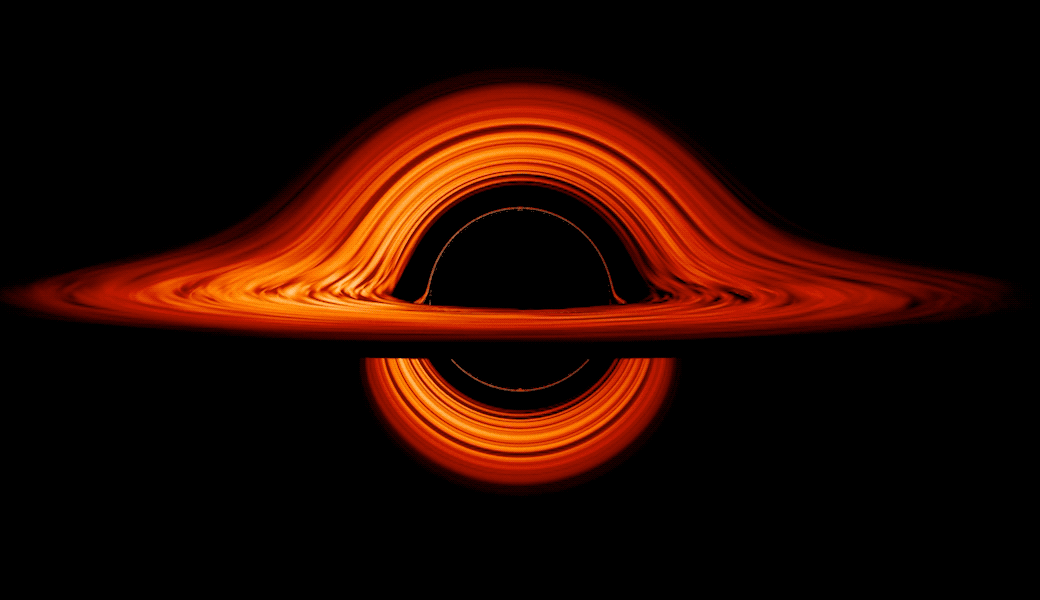
The event horizon is the spherical outer boundary of a black hole loosely considered to be its "surface."
It is the point, according to NASA, that the gravitational influence of the black hole becomes so great that not even light is fast enough to escape it. As a result of the fact that Albert Einstein's theory of special relativity tells us that no signal can exceed the speed of light in a vacuum (c) humanity can never hope to obtain a signal from the one-way boundary that is an event horizon.
As such event horizons effectively act as cosmic gatekeepers preventing us from ever directly observing the secrets that lie at the heart of black holes. Yet they can reveal a great deal about the environment around them.
Related: What happens at the center of a black hole?
"The event horizon is the ultimate prison wall — one can get in but never get out," Avi Loeb, chair of astronomy at Harvard University, told Space.com.
When an item gets near an event horizon, a witness would see the item's image redden and dim as gravity distorted light coming from that item. At the event horizon, this image would effectively fade to invisibility.
Within the event horizon, one would find the black hole's singularity, where previous research suggests all of the object's mass has collapsed to an infinitely dense extent. This means the fabric of space and time around the singularity has also curved to an infinite degree, so the laws of physics as we currently know them break down.
"The event horizon protects us from the unknown physics near a singularity," Loeb said.
Event horizon and black Hole FAQs answered by an expert
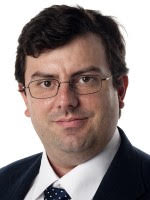
Xavier Calmet is a professor of physics and astronomy in the School of Mathematical and Physical Sciences at the University of Sussex, U.K.
We asked Xavier Calmet, a professor of physics in the United Kingdom a few questions about black holes and event horizons.
What is a black hole event horizon?
It marks the boundary between the black hole and the rest of the universe.
What is special about this boundary?
Nothing inside the horizon can ever escape or come back across this boundary, not even light. This implies that nothing that enters the black hole horizon can be observed from outside this horizon.
Could we ever see past an event horizon?
It is a matter of viewpoint. From that of a distant observer, we never see anything fall into the black hole, i.e., enter the event horizon. The object approaching the event horizon would simply fade away over time and ultimately disappear. However, from the point of view of an observer falling into the black hole, he would not notice anything when crossing the horizon of an astrophysical black hole. Because the curvature at the horizon of astrophysical or large black holes is small, there are no strong tidal forces and the free-falling observer would simply enter the black hole without noticing that he passed the horizon.
What lies past the event horizon?
Empty space and nothing remarkable until one gets very close to the singularity at the center of the black hole. At that stage, tidal forces become extremely strong, and the observer would experience spaghettification — they would be stretched into a long pasta and would eventually be ripped apart by the strong gravitational forces.
Could anything ever escape the event horizon of a black hole?
This is a fascinating question and is linked to the infamous Hawking information paradox. In 2022, along with my colleague Stephen Hsu, we demonstrated in a series of papers how information can escape a black hole. We theorized that information is encoded in the quantum state of the gravitational field outside the black hole and imprinted in Hawking radiation and thus fully available to an outside observer. In that sense, information about what fell into a black hole can always be recovered.
Einstein, black holes, and event horizons: A tale of two singularities
The black hole and event horizon concepts were born from the 1915 theory of gravity conceived by Albert Einstein known as general relativity and solutions to the tensor equations that define it. A tenor is a mathematical equation that is similar to a vector but with four values rather than two. As a result, tensors are also sometimes called "four-vectors."
General relativity is also known as the "geometric theory of gravity" because it says that the presence of mass causes the very fabric of spacetime (the unification of space and time into a 4 dimension entity) to warp.
Think of this as being similar to placing balls of varying masses onto a stretched rubber sheet. The greater the mass of the ball, the more extreme the curvature, so a bowling ball warps the sheet far more than a marble. The same for celestial objects, a star creates more of a warp than a planet. Black holes are created when a massive star completely collapses as a result of its own gravity. The star's mass is compressed into an infinitely small space, causing an extreme warping of spacetime.
Because the term "black hole" refers to that entire warp, not just the infinite mass that dwells at its heart, (Lambourne. R. J. A, 2010, pg 171) it's more accurate to call black holes "spacetime events" rather than objects. And event horizons are the outer edge of these events where spacetime curves so much that anything that passes them is destined to make a one-way trip to the center of the black hole.
In 1915, less than a month after general relativity was first published astrophysicist Karl Schwarzschild became the first person to solve general relativity's field tensor equations, according to the Institute of Physics.
Schwarzschild studied general relativity while serving with the German army on the Eastern Front in the First World War in 191. He wrote to Einstein with his simple and exact solutions which Einstein feared might never be achieved as he himself had only managed approximate solutions. Schwarzschild passed away in 1916, the same year his solutions were published.
The mathematics of general relativity (Lambourne. R. J. A, 2010, pg 171) predicts two singularities. A coordinate singularity, which is the event horizon — the black hole's outer boundary, and a gravitational singularity, which represents the heart of the black hole.
Schwarzschild's solution gave the coordinate singularity and thus the event horizon a solid location around a black hole known as "Schwarzschild radius."
The coordinate singularity can be removed with a clever application of mathematics and transforming the coordinate system. But the gravitational singularity or "real singularity" can't be removed. It challenges physicists to this day, as it represents the point at which the physics that describes the universe completely breaks down.
Where is the event horizon and how big is it?
The Schwarzschild radius (Rs) is a sphere located at the radius from the central "real" singularity of a black hole, which has a radius (r) of r = 0, equal to radius equals 2 times Newton's gravitational constant or "big G" (G) times the mass of the body (M) divided by the speed of light squared (c²) [Lambourne. R. J. A, 2010, pg 172]. The equation for the Schwartzchild radius looks like this Rs = 2GM/c².
That equation implies that the size of the event horizon depends on the black hole's mass. The larger the mass the further out from the central singularity the event horizon will be.
It isn't just black holes that have a Schwarzschild radius all massive bodies like planets and stars do, but these aren't event horizons because these points are usually well within the bodies.
For example, given the sun's mass of 1.989 × 10³⁰ kg, its Schwarzschild radius occurs at about 1.86 miles (3 kilometers) from its central point, that's compared to the sun's radius of around 432,450 miles (696,000 kms). Earth's Schwarzschild radius is even closer to its central point, with our planet having a Schwarzschild radius of no more than 9 millimeters!
To see why the Schwartzchild radius constitutes a light-trapping surface consider that the escape velocity required to race away from the gravitational influence of a body is equal to the square root of 2 times the "big G" (G) times the mass of the body (M) divided by the radius of the body — so Ves = (2GM/r)¹/². For Earth that gives a speed of around 7 miles per second (11.2 kms per second).
For a body with a radius less than 2GM/c² the escape velocity increases to more than 3.0 x 10⁸ meters per second, the speed of light in a vacuum tells us that not even light can outrace gravity at this point.
Another consequence of the Schwartzchild radius is that to become a black hole the radius of a body with mass must shrink to within Rs. If the sun were compressed enough to become a black hole its radius would have to shrink from over 400,000 miles to just 1.86 miles (3 kms). Meanwhile, for our planet to become a black hole the Earth's radius would have to shrink from around 4000 miles (6,400 kms) to just 0.35 inches (0.9 centimeters)!
Sagittarius A*, the supermassive black hole at the center of the Milky Way, is about 4.3 million times the mass of our sun and has a diameter of about 7.9 million miles (12.7 million km), while M87 at the heart of the Virgo A galaxy is about 6 billion solar masses and 11 billion miles (17.7 billion km) wide.
Imaging event horizons
In 2019 the Event Horizon Telescope (EHT) made history by capturing humanity's first direct image of a black hole, or as you'll realize by now more accurately, the environment immediately surrounding the black hole.
The image featured the supermassive black hole at the heart of Messier 87 (M87), located 53 million light-years away with a mass equivalent to around six and a half billion suns according to the EHT collaboration. The image shows a glowing ring of material traveling around the event horizon close to light speed.
The array of telescopes across the globe that comprise the EHT and make an Earth-size virtual telescope made history again in 2022 when it imaged the supermassive black hole at the heart of our own galaxy, known as Sagittarius A* (Sgr A*).
"We were stunned by how well the size of the ring agreed with predictions from Einstein's Theory of General Relativity," EHT Project Scientist and Institute of Astronomy and Astrophysics astronomer Geoffrey Bower said in a statement. "These unprecedented observations have greatly improved our understanding of what happens at the very center of our galaxy, and offer new insights on how these giant black holes interact with their surroundings."
Thus, the image of the black holes at the heart of M87 and the Milky Way demonstrates that while event horizons protect many secrets that lurk behind them, shielding the central singularity at the heart of these spacetime events from scrutiny, they also help reveal a wealth of information about their surroundings and the extreme physics that shape galaxies.
Additional resources
Astrophysicist Karl Schwarzschild is the man who first solved the field tensor equations of general relativity and for whom the location of the event horizon is named. You can read about this extraordinary life in this article published on the science communication website Secrets of the Universe. Read more about the Event Horizon Telescope and the capture of the first black hole image in 2019 with these resources from the Event Horizon Telescope. If you want to learn more about how massive stars die and collapse to form black holes check out these resources from NASA. The agency also explains how it studies these regions of space.
Bibliography
Lambourne. R. J. A., Relativity, Gravitation, and Cosmology, Cambridge University Press, [2010], ISBN: 978-0-521-13138-4 https://www.amazon.co.uk/Relativity-Gravitation-Cosmology-Robert-Lambourne/dp/0521131383
Ta-Pei Cheng, Relativity, Gravitation, and Cosmology: A basic introduction, Oxford University Press, [2010], ISBN 978-0-19-957364
Event Horizon, Swinburne Center for Astrophysics and Supercomputing, [accessed 02/18/23], [https://astronomy.swin.edu.au/cosmos/e/Event+Horizon]
Astronomers Reveal First Image of the Black Hole at the Heart of Our Galaxy, Event Horizon Telescope, [2022], [https://eventhorizontelescope.org/blog/astronomers-reveal-first-image-black-hole-heart-our-galaxy]
Astronomers Image Magnetic Fields at the Edge of M87's Black Hole, Event Horizon Telescope, [2021], [https://eventhorizontelescope.org/blog/astronomers-image-magnetic-fields-edge-m87s-black-hole]
Astronomers Capture First Image of a Black Hole, Event Horizon Telescope, [2019], [https://eventhorizontelescope.org/press-release-april-10-2019-astronomers-capture-first-image-black-hole]
What Are Black Holes? NASA, [accessed 02/18/23], [https://www.nasa.gov/vision/universe/starsgalaxies/black_hole_description.html]
Eisenstaedt, D. Howard, J. Stachel (eds), The Early Interpretation of the Schwarzschild Solution, Einstein and the History of General Relativity: Einstein Studies, Vol. 1, pp. 213-234. Boston: Birkhauser, [1989]
Join our Space Forums to keep talking space on the latest missions, night sky and more! And if you have a news tip, correction or comment, let us know at: community@space.com.
Get the Space.com Newsletter
Breaking space news, the latest updates on rocket launches, skywatching events and more!
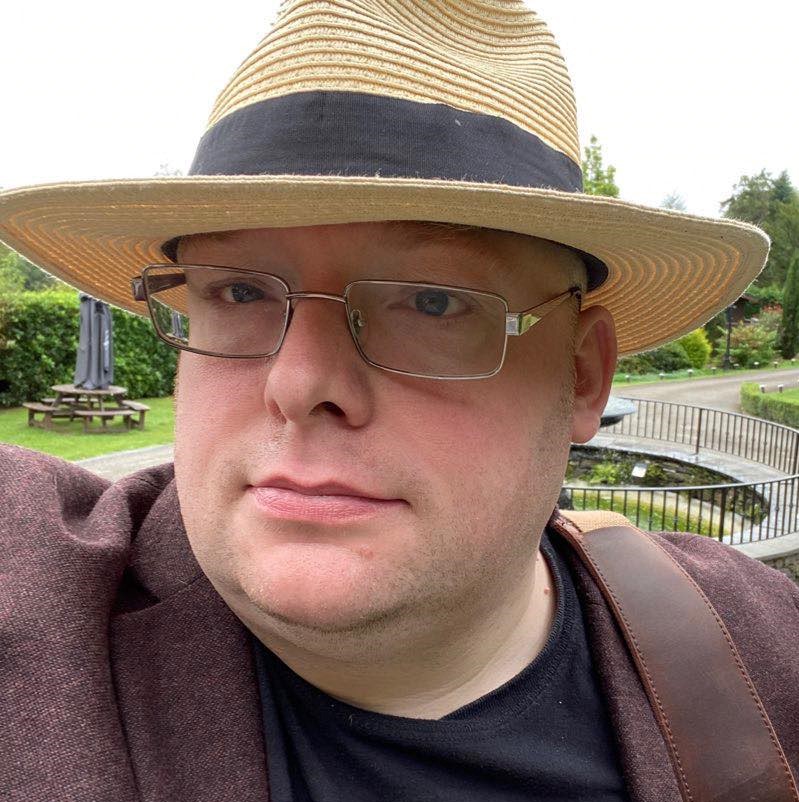
Robert Lea is a science journalist in the U.K. whose articles have been published in Physics World, New Scientist, Astronomy Magazine, All About Space, Newsweek and ZME Science. He also writes about science communication for Elsevier and the European Journal of Physics. Rob holds a bachelor of science degree in physics and astronomy from the U.K.’s Open University. Follow him on Twitter @sciencef1rst.
- Charles Q. ChoiContributing Writer