What are kilonovas?
What happens when the densest objects in the known universe collide?
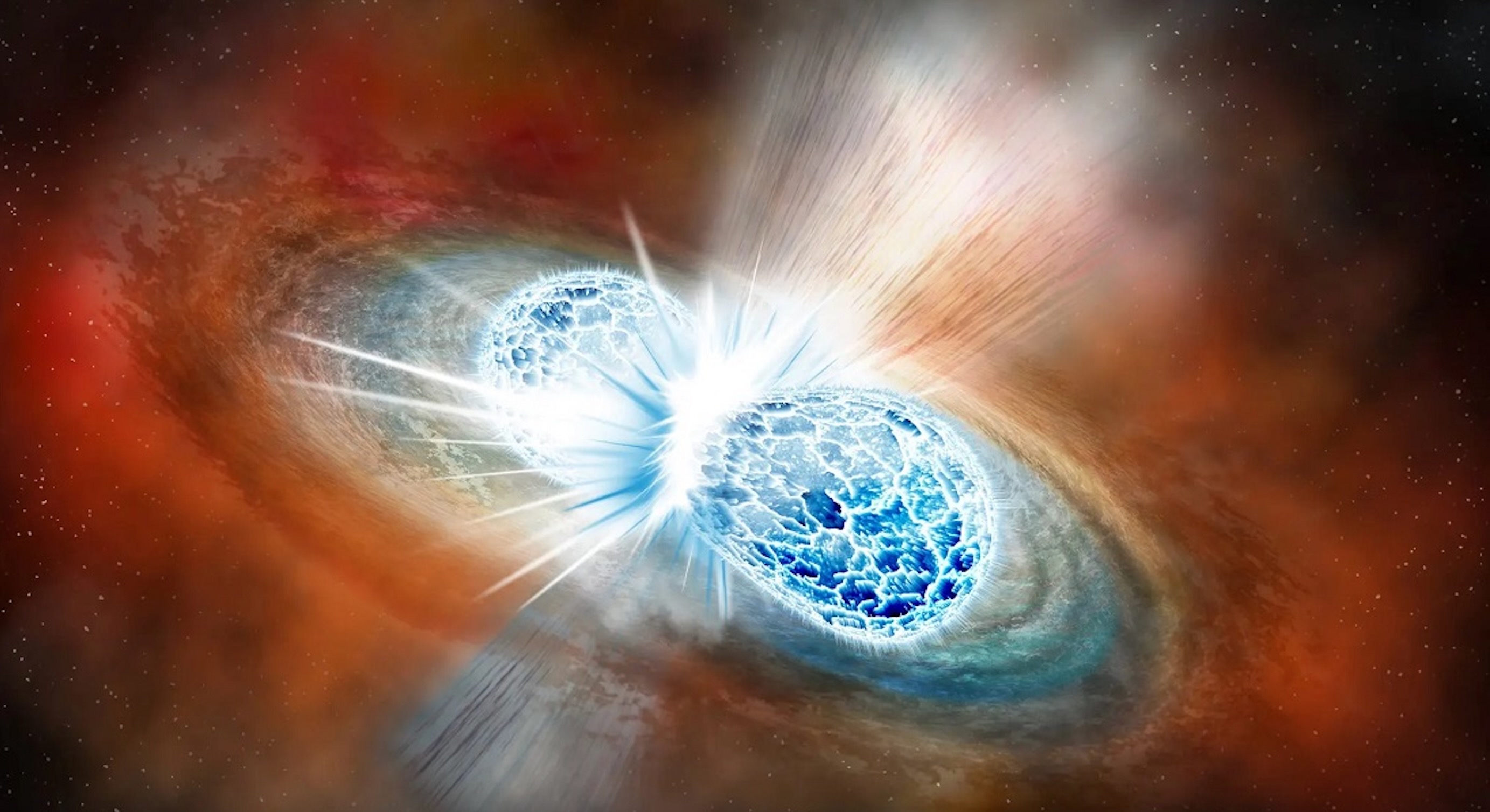
A kilonova is a bright blast of electromagnetic radiation that happens when two neutron stars or a neutron star and a stellar-mass black hole collide and merge.
When these collisions occur, a vast amount of material is ejected from the neutron stars, the ultradense cores of massive stars that have reached the ends of their lives. This matter is rich in neutral particles called neutrons, and in this violent sea of particles around a neutron star merger, the heaviest elements of the periodic table are forged. These include gold and platinum, radioactive materials such as uranium, and the iodine that flows through our blood. In fact, many pieces of jewelry owe their existence to a kilonova-triggering event.
A kilonova is the electromagnetic glow of this ejected material. It is a transient astronomical event, meaning it appears in the sky as a brief flash of light that peaks and then fades before completely disappearing.
Related: Gamma rays: Everything you need to know about these powerful packets of energy
Kilonova expert Q&A
We asked Darach Watson, an associate professor at the Niel Bohr Institute's Cosmic Dawn Center in Denmark, some important kilonova questions.
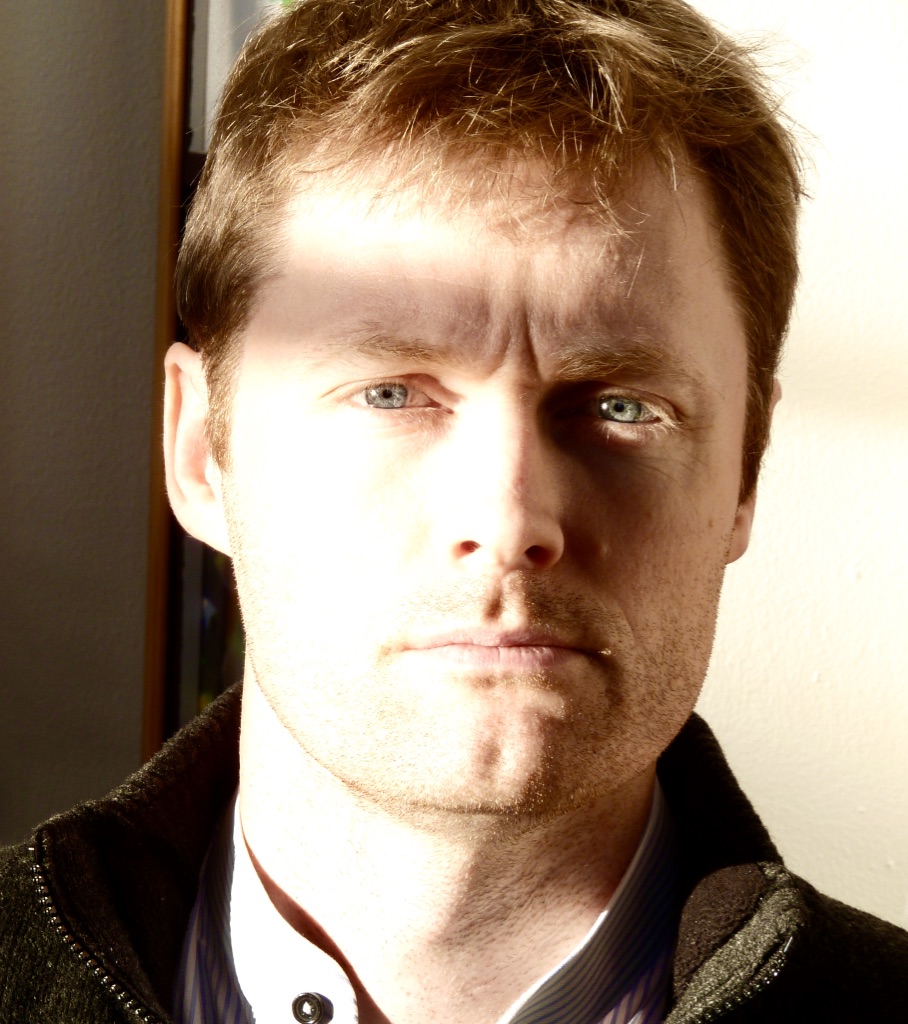
Darach Watson is an astrophysicist at the Niels Bohr Institute. He is a leading expert in X-ray astronomy and has helped to develop our understanding of supernovas, kilonovas and gamma-ray bursts.
What are kilonovas?
When two neutron stars or a neutron star and a black hole collide and merge, they also expel a few percent of their mass. This matter is very special because it is very rich in neutrons and starts immediately to form very heavy elements.
The kilonovae are the astrophysical phenomena we observe of these extremely rapid, radioactive explosions of matter, emitting most of their radiation as optical light.
Why are kilonovas so interesting?
The ejected matter that forms the kilonova is the origin of half of the elements in the periodic table heavier than iron, including many very interesting elements, such as the neodymium in our cell phones, the molybdenum that plants rely on as the central component in the enzyme that allows them to fix nitrogen from the air, or the iodine in our bodies.
By observing kilonovas, we can detect the origin site of the elements and measure how they are formed.
How does your work relate to kilonovas?
We are trying to both detect new kilonovae and to decipher the observations so that we can infer the nuclear physics of how these heavy elements form and the astrophysical circumstances and fundamental particle physics interactions that enable them to be created.
How do you study kilonovas?
We follow up triggers from gravitational wave detectors that tell us that a merger of neutron stars has occurred and try to find its corresponding kilonova in the sky. This is enormously challenging, as they are faint and rapidly fading.
So far, we have only one precious, well-monitored event. The kilonova is observed with large telescopes and monitored spectroscopically so that we can try to determine its composition, geometry and velocity.
What can kilonovas tell us about the universe?
We understand most of the major physical processes that make the chemical elements. Kilonovas can tell us in detail about the last unsolved piece of that puzzle: where the heaviest elements that make up our universe came from. They may also be able to tell us about the interactions of fundamental particles, such as neutrinos, in dense environments, and how matter behaves under super-extreme densities.
I am also optimistic that we may be able to use kilonovae to help us measure the expansion rate of the universe, since some of them at least appear to be extremely accurate standard candles.
What are some of the mysteries surrounding kilonovas currently?
The biggest mystery is how the elements are formed in the merger process — what is the nuclear and particle physics required for that alchemy to occur?
Observationally, we are still at something of a loss as to why our only well-studied event appeared to be spherical. This is quite different from any of the models we have and poses a real challenge.
There is also good evidence for the formation of a narrow jet in the merger process that squeezes out of the merger at close to the speed of light. The origin of that jet is also still something of a mystery.
Finally, we do not know how often neutron star mergers occur and on what timescales, or how much of each element they produce. These questions are key to answering the question of the cosmic origin of the elements.
What's the difference between novas, supernovas and kilonovas?
First suggested in a paper published in 1998, kilonovas were initially referred to as mini-supernovas or "micronovas," since the radiation flashes of neutron star mergers were predicted to be between 1% and 10% as bright as those from typical supernovas.
The term "kilonova" was coined by Brian Metzger, now a professor of physics at Columbia University, in a paper published in 2010, with Metzger and his colleagues pointing out that although a kilonova is less powerful than a supernova, it is still 1,000 times more powerful than a standard nova.
A standard nova happens when a dim stellar corpse called a white dwarf in a binary system pulls matter from a companion star, usually a red giant, causing the white dwarf to suddenly brighten to thousands of times its original luminosity. When this material accumulates on the surface of the white dwarf, it triggers a nuclear explosion that ejects stellar material. The white dwarf begins accumulating matter again from the red giant, and the process repeats.
The word "nova" comes from the Latin for "new" and refers to the fact that stars erupting in novas are too faint to be seen before the sudden brightening, making them appear like new stars in the sky, according to Britannica.
A Type Ia supernova is similar to a standard nova in that a white dwarf strips matter from a binary companion. But in this instance, that flow of material pushes the white dwarf over the mass limit needed to trigger a supernova and the creation of a neutron star.
Type II supernovas also indicate the birth of a neutron star or a black hole. In this case, however, that occurs as the result of the collapse of the core of a massive star at the end of its lifetime. For this reason, these cosmic explosions are often called "core collapse" supernovas, which are more than a million times brighter than novas.
Because it represents light emerging from the collision of neutron stars, a kilonova is clearly different from both a standard nova and a supernova. Yet there is a connection between kilonovas and supernovas, as kilonovas wouldn't be possible without a supernova or two happening first.
What causes a kilonova?
Supernovas are launched when neutron stars or black holes are created, so most kilonovas happen in systems that have experienced two supernovas birth two dense stellar remnants, though the explosive events are likely separated by billions of years.
Specific supernova explosions called ultrastripped supernovas are particularly important to kilonovas because they're vital to the creation of binary systems that can birth a kilonova. These explosive events occur when a companion star strips a neutron star progenitor star of its stellar material, resulting in less material ejected from a dying star than is seen in typical supernova blasts .
Ultrastripped supernova explosions are relatively gentle compared with other supernovas. They also lack the kick velocity needed to throw the forming neutron star away from its binary companion, meaning the neutron star born in such an event can still be orbited by a relatively close companion star.
At this point in the binary system's history, the newly birthed neutron star can start stripping its companion star of its outer layers, reversing the feeding process that led to the first ultrastripped kilonova. Eventually, this triggers a second ultrastripped supernova in the binary system and the creation of a second neutron star, which again ejects little material and lacks the kick velocity to throw it out of its binary system.
The result is a tight binary system in which two neutron stars closely orbit each other, but a kilonova blast is still millions of years away. This spiraling happens because these neutron star binaries — and binaries containing a black hole and a neutron star — constantly emit tiny ripples in space-time called gravitational waves.
The gravitational waves carry angular momentum away from the neutron stars as they orbit each other. As a result, the binary system draws in tighter as its occupants move closer, and it emits gravitational waves at higher and higher frequencies. This causes angular momentum to leak from the system faster and faster, and the neutron stars draw in more rapidly until they collide and merge.
When this kind of collision happens between a neutron star and a black hole, it facilitates the growth of that black hole. When it occurs between two neutron stars, however, it creates a supermassive neutron star, which rapidly collapses to birth a new black hole.
Kilonovas could be precious
Scientists have long understood that the nuclear fusion processes that power stars forge the elements, beginning with the nucleosynthesis of helium from hydrogen, the universe's lightest element.
This creates elements as heavy as iron, with the most massive stars possessing a core of iron when they die in a Type II supernova and leave behind a neutron star or a black hole. The problem with this is that with 26 protons in its atomic nucleus, iron is far from the heaviest element. This is even the case when you don't include non-naturally occurring elements, like 117-proton tennessine, which can be created in the lab.
This meant that the creation of common elements that are heavier than iron — like silver ( 47 protons), gold (79 protons) and platinum ( 78 protons) — had been something of a mystery prior to kilonova theories. Scientists thought these elements could be created via the rapid neutron-capture process, or "-r-process," but for this to proceed stably, it would require an environment absolutely flooded with free neutrons.
Because neutron stars are made almost entirely of a dense soup of neutrons, during collisions, they eject around a gram of neutrons per cubic centimeter into their surroundings. This creates a neutron-rich environment around the merger in which the r-process can proceed.
The r-process begins when the seed nuclei of iron are bombarded with these free neutrons, soaking them up. Stable atoms of iron, such as the isotope iron-56, have 26 protons and 30 neutrons. The excess of neutrons granted to iron by neutron capture makes the iron atoms unstable and radioactive due to a huge imbalance between protons and neutrons. This results in some of the atoms undergoing beta decay, with neutrons transforming into protons via the emission of electrons and other particles like the antimatter equivalent of neutrinos–antineutrinos.
An element is defined by the number of protons in its nucleus, so this process transforms iron into heavier elements, like gold, in what is almost a form of cosmic alchemy. It is the radiation from the decay of the radioactive elements created during the r-process that makes the material ejected from the neutron stars glow , and it's this light that's called a kilonova. Consequently, to understand kilonovas, we must study the creation of heavy elements.
Kilonovas can be identified by their unique observational signature, according to the Harvard and Smithsonian Center for Astrophysics, with these events brighter at near-infrared wavelengths than in visible light. The difference in brightness at these wavelengths is due to the heavy elements ejected by the kilonova, which block visible light while simultaneously allowing the longer-wavelength infrared light to pass through unimpeded.
This means observing kilonovas from Earth is very difficult because only a handful of telescopes, such as the twin Gemini telescopes, are sensitive enough at near-infrared wavelengths to detect these events.
Have we observed any kilonovas?
Mergers between neutron stars and collisions between black holes and neutron stars are responsible for a range of emissions across the electromagnetic spectrum, such as fast radio bursts, short gamma-ray bursts and an X-ray glow. These can be used to locate the violent event that created them, which astronomers use to hunt kilonovas or their afterglow. Early suspected kilonova detections in 2009 and 2013 were associated with short gamma-ray bursts.
Gravitational waves can also be useful in tracking down kilonovas. When spiraling neutron stars finally collide and merge, this creates a sudden blast of gravitational waves that can be detected when they finally reach Earth.
These gravitational waves travel at the speed of light, just like electromagnetic radiation. So when a gravitational wave detector, like the Laser Interferometer Gravitational-Wave Observatory (LIGO), detects gravitational waves from a neutron star merger or a merger between a black hole and a neutron star, astronomers can follow this up to try catching the kilonova in action.
This worked to great effect in August 2017, when LIGO and its fellow gravitational wave detector Virgo detected gravitational waves from a merger of neutron stars located 130 million light-years from Earth. Within 12 hours, the source of the gravitational wave signal — designated GW170817 — was found to be the galaxy NGC 4993, and it was revealed that the kilonova resulting from the merger had been spotted in observations made by the Hubble Space Telescope. GW170817 was the first event to be "heard" in gravitational waves and "seen" with electromagnetic radiation.
In 2022, a team of astronomers spotted what could be a kilonova afterglow that occurred around 3.5 years after the collision and merger of the two neutron stars that created the gravitational wave signal GW170817. This was the result of an X-ray jet from the merger expanding and slowing but leaving behind an X-ray glow with constant brightness.
Astronomers aren't always chasing kilonovas, however; sometimes, they are way ahead of these powerful flashes of light. In early 2023, astronomers saw, for the first time, what they think is a system that is destined to birth a kilonova. The kilonova progenitor system CPD-29 2176 is located 11,400 light-years away and contains two tight, binary objects: a neutron star created in an ultrastripped supernova, and a Be star that the neutron star is stripping of its outer layers and that will eventually become a neutron star via the same process.
It will be some time before the second star triggers one of these rare cosmic explosions. The discoverers of the system told Physics World that the Be star will take millions of years to detonate in an ultrastripped supernova. Even after that, it will take the two neutron stars in the CPD-29 2176 system billions of years to spiral together and merge. The rarity of kilonovas means this progenitor system is a "one-in-ten-billion" observation, its discoverers said in a statement.
As astronomers study kilonovas, these cosmic blasts of light present unexpected qualities that scientists are only just beginning to investigate. For example, in February 2023, scientists found that the kilonova blast associated with GW170817 appears to take on a perfectly spherical shape.
This finding contrasts with all previous models, which suggested that two colliding neutron stars orbiting each other 100 times a second should create an explosion in the shape of a flattened disk. This means kilonovas may be hiding some hitherto undiscovered physics.
Kilonova FAQs
What causes a kilonova?
Kilonovas are bursts of electromagnetic radiation that result from the radioactive decay of iron atoms in material ejected when two neutron stars smash together or when a neutron star merges with a black hole.
Can we see kilonovas?
Yes, we can see kilonovas with telescopes if we know where to look. Neutron star collisions generate gravitational waves, which can be measured on Earth. Astronomers can then attempt to follow up on these detections with telescopes to spot kilonovas.
Unfortunately, gravitational wave detectors aren't good at locating the source of the ripples in space-time they "hear." That means scientists have made only one observation in both gravitational waves and light thus far: the neutron star merger in the galaxy NGC 4993, which was then seen as a kilonova by the Hubble Space Telescope.
What's the difference between novas, supernovas and kilonovas?
All three explosive outbursts of light are caused by different events. Novas are the weakest, with outputs millions of times dimmer than supernovas. Kilonovas are the next strongest, with outputs thousands of times more powerful than novas but still between 10 and 100 times less powerful than supernovas.
How are neutron stars connected to the creation of gold?
The environment around neutron star collisions is rich with free neutrons, which can facilitate a form of nucleosynthesis called the rapid neutron capture process, or r-process. Elements heavier than iron can be created by the r-process when iron, the heaviest element that can be forged by stars, soaks up free neutrons and transforms into other, heavier elements.
Additional resources
A NASA Goddard simulation shows how gravitational wave frequencies increase as neutron stars spiral together and finally merge, resulting in a kilonova. Kilonovas can also happen when neutron stars meet black holes. A video from the Max Planck Institute for Gravitational Physics shows how one of these mixed mergers would proceed. The U.S. Department of Energy explains nucleosynthesis in greater detail.
Bibliography
L-X. Li., B. Paczyński., Transient Events from Neutron Star Mergers, The Astrophysical Journal, [1998], [https://iopscience.iop.org/article/10.1086/311680]
B. Metzger., et al., Electromagnetic counterparts of compact object mergers powered by the radioactive decay of r-process nuclei, Monthly Notices of the Royal Astronomical Society, [2010], [https://academic.oup.com/mnras/article/406/4/2650/1020950?login=false]
F-K. Thielemann., et al., What are the astrophysical sites for the r-process and the production of heavy elements? Progress in Particle and Nuclear Physics, (2011) [https://www.astro.princeton.edu/~burrows/classes/514/papers/thielemann.r-process.sites.pdf]
K.A. Lund., et al, The Influence of β-decay Rates on r-process Observables, The Astrophysical Journal, (2023), [https://iopscience.iop.org/article/10.3847/1538-4357/acaf56.]
Hajela., et al., Evidence for X-Ray Emission in Excess to the Jet-afterglow Decay 3.5 yr after the Binary Neutron Star Merger GW 170817: A New Emission Component, The Astrophysical Journal Letters, (2022), [https://iopscience.iop.org/article/10.3847/2041-8213/ac504a]
J. Rastinejad., et al, A kilonova following a long-duration gamma-ray burst at 350 Mpc, Nature, (2022), [https://www.nature.com/articles/s41586-022-05390-w]
A. Moroianu., et al., An assessment of the association between a fast radio burst and binary neutron star merger. Nature, (2023),[https://www.nature.com/articles/s41550-023-01917-x]
Join our Space Forums to keep talking space on the latest missions, night sky and more! And if you have a news tip, correction or comment, let us know at: community@space.com.
Get the Space.com Newsletter
Breaking space news, the latest updates on rocket launches, skywatching events and more!
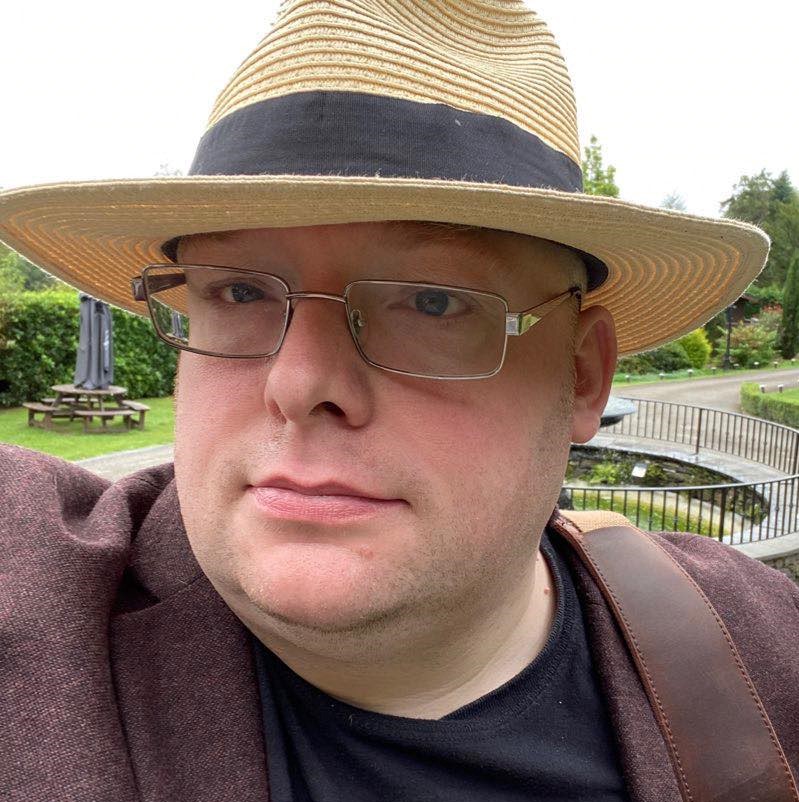
Robert Lea is a science journalist in the U.K. whose articles have been published in Physics World, New Scientist, Astronomy Magazine, All About Space, Newsweek and ZME Science. He also writes about science communication for Elsevier and the European Journal of Physics. Rob holds a bachelor of science degree in physics and astronomy from the U.K.’s Open University. Follow him on Twitter @sciencef1rst.