What are gravitational waves?
These tiny ripples in the fabric of time and space are so faint that even Einstein, the person who first proposed them, believed they would never be detected
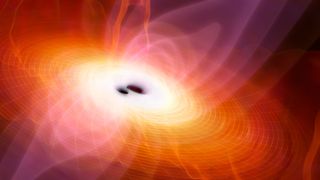
Gravitational waves are tiny ripples in the very fabric of spacetime that propagate outwards from some of the most violent and powerful events in the cosmos. Traveling through the vastness of the universe to reach us here on Earth, these waves gradually lose energy becoming even fainter and more imperceptible.
The measurement of gravitational waves, also known as "gravitational radiation" at facilities such as the Laser Interferometer Gravitational-Wave Observatory (LIGO) operated by the California Institute of Technology (Caltech) and the Massachusetts Institute of Technology (MIT) isn't just significant because it helps to confirm the most accurate theories we have of gravity, one of the universe's fundamental forces, but it offers astronomers an entirely new way to look at the cosmos.
Even more excitingly, observations in gravitational waves can be combined with observations in the electromagnetic spectrum to create a powerful new form of astronomy called multi-messenger astronomy, according to NASA.
Related: Was Einstein wrong? The case against space-time theory
Who discovered gravitational waves?
Gravitational waves were first predicted in Albert Einstein's 1915 theory of gravity, known as general relativity. One of the main conclusions of this theory is that gravity emerges as a result of the fact that objects of mass warp the very fabric of spacetime.
This is analogous to taking a stretched rubber sheet and placing balls of different masses upon it creating "warps" in its fabric. The bigger the mass of the ball the more extreme the warp it causes.
Objects sat in spacetime are similar but in three dimensions of space and one dimension of time rather than the two dimensions of a stretched rubber sheet. The greater the mass of an object the more extreme the warping of space it causes, so a star warps spacetime more than a planet, and a black hole warps it more than a star.
General relativity goes further than this, however, when describing the effect of mass on spacetime. LIGO explains that the mathematics of Einstein's theory predicted that two accelerating objects of great mass should disrupt spacetime causing it to undulate and sending waves propagating outwards from it.
These ripples in spacetime, spread out in all directions from their source, traveling at the speed of light, which also happens to be the speed of gravity. Carried by these gravitational waves and encoded within them is information about the events and objects that created them and sent them through the cosmos.
Gravitational waves FAQs answered by an expert
We asked Elenna Capote, LIGO Scientific Collaboration fellow working at the LIGO Hanford Observatory some questions about gravitational waves.
Elena Capote is a LIGO Scientific Collaboration fellow working at the LIGO Hanford Observatory.
What are gravitational waves?
Gravitational waves are ripples in spacetime. These ripples occur when mass accelerates. The larger the mass or the faster the acceleration, the stronger the gravitational wave.
What types of events create these waves?
All gravitational systems can create gravitational waves. However, gravitational waves are very weak. Even Einstein believed it would be nearly impossible to detect these waves. Therefore, the events we look for are the events that make the strongest gravitational waves in the universe. These events are things like compact binary coalescences, such as merging black holes or neutron stars, exploding stars such as supernovas, fast-spinning neutron stars like pulsars, or the leftover gravitational wave background from the big bang. Even more exciting than these already fascinating events is the possibility of the unexpected. Exotic predictions like cosmic strings, dark matter, or something that we haven't even predicted could create a gravitational wave.
How fast do they travel across the universe?
Gravitational waves travel at the speed of gravity! It is through the work of LIGO, Virgo, and observations from other astronomers that we learned that the speed of gravity is equal to the speed of light. Einstein predicted this, but our exciting detections teach us that this prediction was correct.
How do we detect gravitational waves here on Earth?
We detect gravitational waves by using a 2.5 mile (4 kilometer) detector with two "arms". The detector is what we call a Michelson Interferometer (named after the scientist that developed this technology).
The Michelson Interferometer uses a laser beam that is split so that the laser light travels down each arm, reflects off mirrors at the end of each arm, and returns to the output where the beams are recombined. The detector arms are set to be the same length, however, if a gravitational wave stretches and squeezes the spacetime in one direction, the two beams will interfere with each other because they will be out of phase. The signal that we see at the output will change based on that interference, telling us the properties of the gravitational wave.
What can we learn about distant objects and events using gravitational waves?
Gravitational waves encode information about the events that create them. The frequency of the wave will depend on the masses of the objects, and the strength will depend on how far away the event occurs. By fitting the gravitational wave signal, we can determine these parameters and other characteristics of the system, such as the spin of the masses or the eccentricity of the orbits. With gravitational waves, we have learned about the physics that governs matter at the highest densities and that black holes collide much more often than we thought.
How does your work relate to gravitational waves?
I work on gravitational wave detector commissioning. Detector commissioning is, in essence, making the instrument work. A gravitational wave detector has many components, and commissioning them requires an understanding of how these components interact so that the detector can do its primary job: detect gravitational waves. As a part of my job, I develop control loops for various systems such as mirror suspensions, work with lasers and optical systems, and make precision measurements to define detector sensitivity.
Why do gravitational waves reveal things traditional astronomy can't?
Some gravitational waves are emitted from events that do not produce light. These events are not witnessed by traditional astronomy because traditional astronomy depends on light signals. With gravitational wave detection, we get a whole new perspective of the universe and the kind of objects that exist in it. Even detecting gravitational waves from events that produce light, such as neutron star mergers, is useful. We get information about the merger before the collision occurs and the light is released. We can make better constraints on the parameters of the merger, and link theoretical ideas about mergers with actual data.
What kind of events create gravitational waves?
According to LIGO, any object with mass that accelerates creates gravitational waves including Earth-based objects like airplanes, cars, and even humans. But the masses associated with these objects and the relatively slow speeds at which they accelerate means the gravitational waves they create are far too weak to ever be detected.
To spot gravitational waves, astronomers and physicists need to look toward objects far greater in mass than even the sun. Fortunately, the universe beyond the solar system is filled with massive accelerating objects.
Events such as the orbit of two black holes or neutron stars, both types of objects born when massive stars run out of fuel for nuclear fusion and collapse under their own gravity, create gravitational waves that can be measured on Earth.
As these objects spiral around each other they send sending gravitational waves propagating outwards carrying away energy, according to LIGO. This causes the black holes or neutron stars or even a combination of the two to collide. These cataclysmic mergers release 50 times more energy than all the stars in the observable universe, but last only for fractions of a second.
These collisions also create the strongest gravitational waves, so strong that even after traveling billions of light years and losing energy along the way, they can still be detected here on Earth. According to LIGO, by the time gravitational waves created by black hole mergers reach Earth, they can be thousands of billions of times smaller than when they first began their journey.
Gravitational waves can also be emitted alongside supernovas during the gravitational collapse of massive stars to create neutron stars and black holes. They are also created by the rapid rotation of neutron stars due to the fact their surfaces are not completely even. These different events and objects give rise to different types of gravitational waves.
Types of gravitational waves
According to LIGO, there are four different types of gravitational waves that scientists search for in data from the facility.
Compact binary inspiral gravitational waves
Compact binary inspiral gravitational waves are created by orbiting pairs of dense massive objects like black holes, neutron stars, and even white dwarf stars. The combination of objects in the pairing creates a unique gravitational wave pattern. Despite this, the generation mechanism for this class of gravitational waves is the same no matter the source.
Known as "inspiral" this process occurs over millions of years as the dense objects revolve around each other moving closer and closer as energy is carried away by gravitational waves. As this happens the objects revolve around each other faster generating stronger and stronger gravitational waves. As result, they lose energy and are drawn together even more rapidly increasing the frequency and amplitude of the gravitational waves they create until they are within the range frequencies of LIGO and other interferometers can "hear."
How long compact binaries can give off gravitational waves is dependent on the mass of the objects that make up the binary, according to LIGO. This is because the most massive objects like black holes move through the inspiral binary stage more rapidly.
LIGO illustrates this by pointing out that the gravitational wave signal from the first detected inspiral black hole pair lasted just two-tenths of a second long, while the first signal from a neutron star-neutron star binary lasted 100 seconds long.
Continuous gravitational waves
Continuous gravitational waves are believed to be created by the rotation of massive single objects like neutron stars as a result of bumps and deformations at their surface when the spin rate of the object remains the same. When shown as a wave, continuous gravitational waves from a single object would appear to have stable frequencies and amplitudes. LIGO points out that continuous gravitational waves from a single object are yet to be detected as current detectors aren't sensitive enough to do this.
Stochastic gravitational waves
Stochastic gravitational waves describe small gravitational waves from less massive objects constantly washing over Earth from every direction all over the universe. These weak gravitational waves are randomly mixed together creating a "stochastic signal" — a random signal that can be statistically analyzed but not predicted.
As the smallest gravitational wave signals, stochastic gravitational waves should also be the most difficult to detect. LIGO predicts that the Big Bang may have left behind "fossil" gravitational waves in the form of stochastic gravitational waves and if we can ever develop instrumentation sensitive enough to detect them these primordial ripples in spacetime could allow us to look further back into the history of the universe than any other form of astronomy.
Burst gravitational waves
Burst gravitational waves are short in duration and come from unknown sources. Described by LIGO as gravitational waves that "go bump in the night" these gravitational waves are expected to come from systems that astronomers haven't yet spotted. Current theories suggest that these gravitational waves are created by events that also trigger supernovae or gamma-ray bursts.
"Searching for burst gravitational waves requires being utterly open-minded. For these kinds of gravitational waves, scientists must recognize a pattern of signals even when such a pattern has not been modeled (what we think a signal may look like) before," LIGO writes. "If you don't know what you're looking for, it's really hard to find it. While this makes searching for burst gravitational waves difficult, detecting them has the greatest potential to reveal revolutionary information about the universe."
Who measured gravitational waves first?
Twenty years after the death of Einstein in 1954, physicists Russell Hulse and Joseph Taylor used the Arecibo Radio Observatory in Puerto Rico to discover a tantalizing hint at gravitational waves in the form of a pair of rapidly spinning neutron stars or pulsars.
Knowing a pulsar should emit gravitational waves robbing the binary pulsars of energy and forcing their orbits together, the duo tested Einstein's theory of general relativity by watching the binary pulsars for eight years.
This revealed that the orbits of the pulsars tightened by precisely the amount predicted by general relativity if they were emitting gravitational waves. The discovery would earn Hulse and Taylor the 1993 Nobel Prize in Physics.
The first direct observation of gravitational waves came on September 14, 2015, 100 years after the publication of Einstein's theory of general relativity. The twin LIGO interferometers, located in Livingston, Louisiana, and Hanford, Washington observed gravitational waves from the merger of two black holes, each about 30 times the mass of our sun. The signal from the event designated GW150914, with the "GW" prefix standing for "Gravitational Wave," was detected at 5:51 a.m. Eastern Daylight Time (09:51 UTC), according to a statement from LIGO announcing the breakthrough issued on Feb. 11, 2016.
According to Matthew Evans, assistant professor of physics at Massachusetts Insitute of Technology, at that time a century earlier even Einstein had predicted gravitational waves would be too faint to ever be detected.
"The effect we're measuring on Earth is equivalent to measuring the distance to the closest star, Alpha Centauri, to within a few microns," Evans said in a statement. "It's a very tough measurement to make. Einstein expected this to never have been pulled off."
As of November 2021, the LIGO-Virgo-KAGRA collaboration has detected at least 90 gravitational wave signals from a variety of different events. This included the first detection by LIGO and Virgo of a neutron star-neutron star merger on August 17, 2017, designated GW170817.
This was followed by the first and second detection of gravitational waves from a so-called "mixed merger" consisting of a black hole colliding with and swallowing a neutron star. The events designated GW200105 and GW200115 were picked up just ten days apart on Jan. 5, 2020, and Jan. 15, 2020, respectively.
The event that created this first detected gravitational wave signal was designated as GW150914 and according to LIGO the "wobble" in spacetime it created was 1000 times smaller than the nucleus of an atom, demonstrating how incredibly sensitive these detectors actually need to be.
How are gravitational waves detected?
As the name suggests gravitational wave observatories are responsible for detecting these tiny ripples in spacetime when they reach Earth. Though LIGO is arguably the most famous of these observatories as the home of the first direct observation of gravitational waves, scientists have been working towards gravitational wave detectors since the 1960s.
The modern approach of using laser interferometry to detect gravitational waves that form the foundation of LIGO's two detectors, as well as the Virgo detector in Italy, and the Kamioka Gravitational Wave Detector (KAGRA) in Japan, was first pioneered in 1962. The first interferometric gravitational wave detector was invented ten years later in 1972 by American physicist Rainer Weiss who would go on to help found LIGO.
Laser interferometers date back much further than the concept of gravitational waves, all the way back to the 1800s in fact, and were famously used in the infamous Michelson-Morley experiment to detect the so-called "luminiferous ether"— the medium scientists once believed light travels through.
Though taking a wide range of shapes when used in a variety of scientific investigations the interferometers used to detect gravitational waves consist of a beam splitter, two laser "arms," and a photodetector. This latter component measures an interference pattern when the beams of light come back together.
This inference pattern is caused by the meeting of peaks and troughs in the wavelengths of light. Where two peaks or two troughs meet a larger peak is created which is the sum of the two peaks — total constructive interference — but where a peak meets a trough the features cancel each other out — total destructive interference. The key takeaway is the interference pattern is always the sum of the height of the peaks and the depth of the troughs that meet with partial constructive or destructive interference also possible.
Related: The double-slit experiment: Is light a wave or a particle?
The laser arms of gravitational wave detectors like LIGO are purposefully designed so that when beams of light meet there is total destructive interference. This is only if the beams of light travel exactly the same distance. But, if one of the beams of light for some reason travels a slightly longer distance the light is no longer in sync and there is no longer total destructive interference. As ripples in spacetime cause space itself to stretch if a gravitational wave travels over a beam arm it can cause that beam to travel an infinitesimally small distance more than its partner beam. This results in partial interference and the photodetector picking up a tiny flicker of light.
According to LIGO the change in length of a beam arm caused by a gravitational wave can be as small as 1/10,000th the width of a proton or 0.0000000000000000001 meters! The tiny flickers of light that indicate a gravitational wave passing also have to be distinguished from the other flickers that a laser interferometer experiences that can be caused by a range of very down-to-Earth phenomena like earthquakes or even passing traffic.
Earth's main gravitational wave detectors, LIGO, Virgo, and KAGRA all use laser interferometers on a massive scale. For instance, the laser arms of LIGO are 2.5 miles (4 kilometers) long and compose the largest laser interferometer ever built. For comparison, the arms of the laser interferometer used in the Michelson-Morley aether hunting experiment were just 4.3 ft (1.3 meters) long.
LIGO is sensitive enough to detect the smash-up between massive black holes and neutron stars, but NASA is currently planning a more sensitive space-based gravitational wave detector called LISA (Laser Interferometer Space Antenna). This should be sensitive enough to detect ripples in spacetime from much smaller systems like relatively small stellar mass black holes orbiting supermassive black holes.
Ringing spacetime: Do gravitational waves make a sound?
The way gravitational waves propagate also seems analogous to the sound waves, but in the near vacuum of space where there isn't a medium like air there can't be sound so gravitational waves remain silent.
However, when gravitational waves are detected here on Earth their frequencies can be converted into sound thus enabling us to hear the "ringing of spacetime" that is created from the collision and merger of black holes or neutron stars, or a mix of the two.
LIGO converted the frequency of the gravitational waves detected on September 14, 2015, from the merger of two black holes, each about 30 times the mass of our sun to sound. This allowed us to "hear" the ringing of spacetime created by two colliding cosmic titans.
As the black holes spiral closer together the frequency of the gravitational waves increases creating increasing the frequency of the gravitational waves which when converted to sound resemble a bird's "chirp."
Multimessenger astronomy: A new window on the universe
As well as being an incredible discovery in itself the detection of gravitational waves has provided astronomers with a way of looking at the universe via gravitational wave astronomy.
Astronomers have traditionally used the electromagnetic spectrum and its range of frequencies from low-energy ultraviolet light through visible light to high-energy gamma rays as the basis of astronomy, but objects like black holes and dark matter that do not emit light remain invisible to this form of astronomy.
Additionally, while electromagnetic radiation interacts strongly with matter, being absorbed, reflected, refracted, or bent as it travels the cosmos, gravitational waves only interact with matter weakly. LIGO says that as a result of weak interaction these ripples in spacetime travel great distances across the universe unimpeded and free of distortions.
These gravitational waves, therefore, carry uncorrupted information about the incredibly distant events that created them allowing astronomers to "hear" phenomena in the universe that conventional astronomy can't "see."
Some events heard in gravitational waves that do emit light can then be followed up using investigations in the electromagnetic spectrum, forming a powerful new form of astronomy called "multimessenger astronomy." This can also include detections of ghost-like particles called neutrinos and high-energy charged particles called cosmic rays, according to NASA.
The neutron star-neutron star merger GW170817 is an example of the potential of multimessenger astronomy. Following the detection of this event in gravitational waves astronomers observed it using electromagnetic waves.
The light from this merger came in the form of gamma rays that followed two seconds after the gravitational waves, then X-ray, ultraviolet, optical, infrared, and radio waves emissions followed for days and weeks after the merger. This allowed scientists to track this event back to its home in the old elliptical galaxy 130 million light-years from Earth.
According to Havard and Smithsonian Center for Astrophysics multimessenger astronomy can be used by scientists to probe the structure of neutron stars and to investigate the powerful collisions between these stellar remnants that are believed to create heavy elements like gold.
Additional resources
An animation created by LIGO at Caltech shows spiraling black holes heading towards a merger that will create a massive black hole with a mass 21 times that of the sun. The future of gravitational wave astronomy is space-based. Learn more about NASA's proposed space-based laser interferometer and gravitational wave observatory LISA, with these resources from NASA. Read more about the detection of gravitational waves in this explainer article published on the University of Birmingham's website.
Bibliography
M. Maggiore, Gravitational Waves Volume 1: Theory and Experiments, Oxford University Press, 2019, ISBN 978-0-19-857074-5
M. Maggiore, Gravitational Waves Volume 2: Astrophysics and Cosmology, Oxford University Press, 2019, ISBN 978-0-19-857074-9
What are Gravitational Waves, LIGO Caltech, [Accessed 03/17/23], [https://www.ligo.caltech.edu/page/what-are-gw]
LIGO- A Gravitational-Wave Interferometer, LIGO Caltech, [Accessed 03/17/23], [https://www.ligo.caltech.edu/page/ligo-gw-interferometer]
Gravitational Waves, NASA Hubblesite, [Accessed 03/17/23], [https://hubblesite.org/contents/articles/gravitational-waves]
[https://www.ligo.org/detections.php]
A. Neronov., Introduction to multimessenger astronomy, arXiv, [2019],
[https://doi.org/10.48550/arXiv.1907.07392]
Multimessenger Astrophysics Science Analysis Group, NASA, [Accessed 03/17/23], [https://pcos.gsfc.nasa.gov/sags/mmasag.php]`
LIGO, Britannica, [Acessed 03/17/23], [https://www.britannica.com/topic/Laser-Interferometer-Gravitational-wave-Observatory]
Thorne. K., R. Weiss., A Brief History of LIGO, Caltech, [Accessed 03/17/23], [https://www.caltech.edu/about/news/brief-history-ligo]
Join our Space Forums to keep talking space on the latest missions, night sky and more! And if you have a news tip, correction or comment, let us know at: community@space.com.
Get the Space.com Newsletter
Breaking space news, the latest updates on rocket launches, skywatching events and more!
Robert Lea is a science journalist in the U.K. whose articles have been published in Physics World, New Scientist, Astronomy Magazine, All About Space, Newsweek and ZME Science. He also writes about science communication for Elsevier and the European Journal of Physics. Rob holds a bachelor of science degree in physics and astronomy from the U.K.’s Open University. Follow him on Twitter @sciencef1rst.