
On the Origin of Worlds: Astrophysicists Zero in on How Planets Form (Kavli Roundtable)
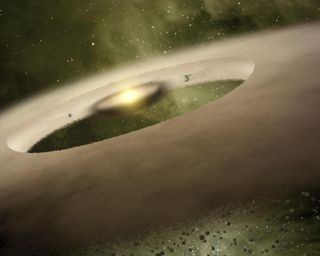
Adam Hadhazy is a writer and editor for The Kavli Foundation. You can read more from the foundation and their researchers on their Kavli Foundation Expert Voices archive page. Hadhazy contributed this article to Space.com's Expert Voices: Op-Ed & Insights.
The secrets of planet formation are becoming harder to keep. In November, using a new observing method, scientists snapped the very first pictures of an extrasolar planet still gathering up mass from its dusty, planetary nursery. Called LkCa 15 b, this immature gas giant has opened a window into the poorly understood process of how planets form.
LkCa 15 b came on the heels of the discovery of 51 Eri b, a relatively adolescent world of 20 million years that, at this point in its development, is like a young Jupiter. Though fully formed, 51 Eri b is still radiating heat from its tumultuous birth. This leftover heat provides tantalizing clues about the conditions in which 51 Eri b has grown up.
The discovery of both these exoplanets was made possible by a technique called "direct imaging," wherein the faint light from a planet is separated from the overwhelming glare of its host star. Compared to other methods, direct imaging lets astronomers more readily study the atmospheric composition of a newly formed (or even forming) world, providing key insights into these objects' ultimate origins.
On January 20, 2015, The Kavli Foundation spoke with three planetary formation experts. The discussion covered promising new ways of studying how giant planets form and whether they can explain the rise of our entire Solar System.
The participants were:
Bruce Macintosh, a professor of physics at Stanford University and a member of the Kavli Institute for Particle Astrophysics and Cosmology (KIPAC). Macintosh is the Principal Investigator of the Gemini Planet Imager project that discovered 51 Eri b, announced in the journal Science.
Get the Space.com Newsletter
Breaking space news, the latest updates on rocket launches, skywatching events and more!
Kate Follette is a postdoctoral researcher, also at KIPAC at Stanford University. She is the co-lead author on a Nature paper on LkCa 15 b and co-author of the 51 Eri b study.
Ruth Murray-Clay is an assistant professor of physics at the University of California, Santa Barbara, and also a co-author of the 51 Eri b study.
The following is an edited transcript of their roundtable discussion. The participants have been provided the opportunity to amend or edit their remarks.
The Kavli Foundation: How are the discoveries of 51 Eri b and LkCa 15 b helping to complete a "family album" of how giant planets form and develop?
Bruce Macintosh: In the family album, so to speak, 51 Eri b is as big as it's going to get, like an adolescent, but still kind of angry, warm and turbulent, again like an adolescent. One could set up the analogy that objects in protoplanetary disks, like LkCa 15 b, are still in utero. But LkCa 15 b already has a solid core. It's formed, which is sort of birth-like, and now it's in the process of growing. It's accreting mass and growing like a child.
Kate Follette: The planets, such as 51 Eri b, that we image with the Gemini Planet Imager are indeed like turbulent adolescents. They are fully formed, but in terms of their age as a proportion of the total lifetime of their system, which is billions of years, they're just barely out of the womb.
Macintosh: We could say that planets age like dogs. [Laughter] They don't age on a linear scale with people.
Ruth Murray-Clay: We've seen a lot of gas giant planets in observational surveys, like from the Kepler space telescope. But these giant planets tend to be very close to their stars. We have a standard picture that gas giants form at distances from their stars where Jupiter and Saturn are found in our Solar System. 51 Eri b doesn't fit that picture. It's a relatively cool world at a more Jupiter-like distance from its star, and we had never seen that before. It suggests that some of these giants must dynamically move closer to their stars as their solar systems evolve. We'd really like to know if that is the right way of thinking about the overall architecture of planetary systems, and 51 Eri b is the start of that.
With regard to LkCa 15 b, from a planet formation perspective it's incredibly exciting to be seeing a planet possibly still in the process of accumulating mass, or accretion. It's very difficult to model that process theoretically, partly because we just don't know how accretion happens in young disks and onto planets. So having any sort of observational constraints on those theories like we're getting by studying LkCa 15 b will take us a long way.
TKF: To see a baby planet still accreting material, Kate and her colleagues turned to a new method to study LkCa 15 b. Using the Magellan telescope in Chile, they detected the signature of heated, ionized hydrogen gas — called "H-alpha" — as the gas fell from a protoplanetary disk onto a world just taking shape. Kate, will you be able to use this method to study other planets still taking form?
Follette: We certainly hope so. We have a sample of about 20 more objects that we think are good candidates for protoplanets similar to LkCa 15 b because they are in so-called transition disks. These are protoplanetary disks that have big, central cavities that are cleared of all of the gas and dust that usually surrounds a young star. The youngest protoplanetary disks are shaped like pancakes, with material reaching all the way in to the star in the middle. Transition disks, on the other hand, are like donuts, and researchers have long postulated that their central holes are cleared by the gravitational influence of planets. The LkCa 15 b image is significant because it is the first time we've been able to show this to be true by directly imaging a planet inside a disk gap.
We know that the central stars in these disks are often still actively accreting material from the disk. Therefore, gas and dust must somehow be making it from the outer disk onto the star, and so you can logically conclude that this material is also falling onto intervening planets. At the same time, because the cavities are cleared of material, we can see all the way down to the mid-plane of the disk — sort of like the "midline" on a donut, if you will — where we think the planets are forming.
Given this setup, we really think transition disks and accreting planets are a sweet spot for this particular type of imaging. The planet is glowing so brightly in H-alpha, it means that we don't have to work as hard to isolate its light emission as we would otherwise when trying to study a dim planet near a bright star. As a result, we can work even closer to the star, all the way to the inside of these disk gaps. Just as a comparison, when Bruce, Ruth and I worked on the 51 Eri b discovery, its star was about 500,000 times brighter than 51 Eri b. The light that my colleagues and I isolated from LkCa 15 b is only a few hundred times fainter than its star.
Macintosh: That's like cheating. That's too easy! [Laughter]
Follette: Yeah, exactly!
TKF: Could cracking the case of how giant planets form potentially trickle down to understanding how Neptune-size and even rocky, Earth-like worlds form?
Murray-Clay: The current dominant model of how gas giants form is that a solid, rock and ice core forms first. The core becomes massive enough to accrete gas from the surrounding protoplanetary disk. With that model in mind, one way of thinking about Neptunes or even Earths is as planets that failed to grow massive enough to become gas giants. For a Neptune, you can ask if it were massive enough to accrete a Saturn-sized envelope, why didn't it? Why did it fail? And for an Earth, you can ask why wasn't there enough material around for it as a rocky core to grow big enough to become a gas giant instead?
The theoretical answers to those questions typically depend on how much available mass there is in a protoplanetary disk. Other considerations are how disturbed the disk becomes over time, which affects how long it takes rocky cores to grow, and whether that process takes too long to form gas giants compared to the lifetime of the dissipating gas disk.
If the basic ideas that many of us astrophysicists share about planet formation are correct, then those ideas should tell us something about the kinds of architectures that we'd expect to find in planetary systems. If you want to understand why you have an Earth in a solar system, you also need to understand why you don't have a Jupiter.
Macintosh: To understand planetary formation processes, a lot of people have tried "archaeological" approaches. This is where you look at the statistics of the planets discovered in mature solar systems and compare these statistics to the predictions from various planetary formation paths. The history of success of that approach is relatively weak, though. It's been very hard to develop a model that successfully predicts the new solar systems we keep discovering.
TKF: Do we have a limited or skewed view of planetary formation because most theories are based on the single example of our own Solar System? After all, we did not even know about another multi-planet system around a Sun-like star until 1999.
Follette: We are discovering planets that are very unlike what we see in our Solar System, but then again, the field of exoplanetology is so young. The main techniques we have been using to discover exoplanets favor high-mass planets very close to their stars, like for instance the so-called "hot Jupiter" class of worlds. Maybe they are the outliers. They might just be the easy-to-detect, oddball planets of the universe. So the fact that we found a lot of hot Jupiters or closely packed planetary systems may not be indicative of what the norm is in the universe.
Murray-Clay: I agree with that. It may be that we're actually being sent down the wrong path by the exoplanet discoveries we're making, not the other way around by starting with our Solar System. Now that can't entirely be true, because from the number of solar systems that Kepler has seen, we can infer that something like 30 percent of stars host "super-Earths." So super-Earths, which have anywhere from two to 10 times the mass of Earth and which we don't have in our Solar System, are clearly a common outcome of planet formation. However, we also don't know what the solar systems seen by Kepler look like in their entirety, because Kepler's data cannot really tell us about any planets in more distant orbits. We really don't know that Solar System-like architectures are not common.
TKF: What are some promising planetary formation models or explanations, then, that would account not only for our Solar System, but those strikingly different solar systems we have glimpsed in recent years?
Murray-Clay: It may be that there's a range of solar system masses. Very high-mass systems may be more likely to produce gas giants. Those gas giants are more likely to be gravitationally kicked into their inner solar system and therefore be easily observable by our conventional exoplanet detection techniques, like the "radial velocity method" as well as the "transit method" seen by Kepler.
Then you get to a little bit lower mass solar systems and you get something like our Solar System. You have some gas giants in this scenario, but they didn't get kicked around very much to inner or outer orbits by the gravity of other planets in the system. Then, you go to even lower mass systems, and there you might end up with systems that have no gas giants at all and just a number of Earths and mini-Neptunes. Within a framework like that, the Solar System is just part of a spectrum of possible solar systems.
Macintosh: To some extent, though, it seems like it's getting harder to have a continuum for solar system architectures that includes our Solar System and the Kepler-discovered solar systems. There are not a lot of intermediate solar systems. You kind of end up wanting a more bimodal or trimodal process, where the possible solar system outcomes branch instead of there being a range of possibilities.
Murray-Clay: But how would we know that yet? We're only really seeing the very inner parts of some solar systems with Kepler.
Macintosh: That's certainly true, but a system like ours with orbits scaled down by 30 percent, or planet sizes scaled up by 30 percent, would be fairly detectable by Kepler.
Murray-Clay: There's a lot we don't know yet, though we can say that a large fraction of stars have solar systems that are not like ours. I just think it's still not clear that the Solar System is uncommon.
TKF: Complicating matters further, researchers at Caltech, including Kavli Prize-laureate Mike Brown, recently presented theoretical evidence for a new, super-Earth-size world lurking way out in the Kuiper Belt, far beyond Pluto. Could this "Planet Nine," if it's real, scramble our prevailing Solar System planet formation models?
Macintosh: Positing that Planet Nine does exist for the sake of discussion, it's not necessarily crazy compared to what we see in other solar systems. We were just discussing that the lack of super-Earths in our Solar System is one way we differ from the majority of the solar systems discovered by the Kepler mission. Now we might have this far-out super-Earth called Planet Nine, but not correspondingly where Kepler sees these sorts of planets in other solar systems. So you need a mechanism to explain how Planet Nine got way out there.
We know from observations of giant planets that they can get scattered from the inner parts of solar systems to the outer parts. One example is the HR 8799 system, which has four Jupiter-size worlds. Two of them are orbiting farther out than Neptune. Putting those ideas together that, A, our Solar System could have formed a super-Earth-slash-mini-Neptune; and B, that you could move that super-Earth-slash-mini-Neptune very far out is surprisingly not insane by astronomer's standards.
Murray-Clay: I completely agree with Bruce — not insane at all. Kicking things out to large orbits is a reasonably common outcome. I'll be very excited if they find Planet Nine, but I don't think it would necessarily change our view of the formation of the Solar System that much.
TKF: What upcoming projects and instruments are you all looking forward to that could help further unravel the mysteries of planet formation? Bruce, you were just selected to lead an exoplanet team for NASA's next major astrophysics observatory, the Wide Field Infrared Survey Telescope (WFIRST). The direct imaging of exoplanets could be a prime mission for this future telescope, slated for launch in the mid-2020s. Why should we be excited?
Macintosh: The most interesting thing with WFIRST will be the ability to characterize the make-up of planets. Analyzing the light that comes from planets can tell you about their composition, which helps you understand how much mass they accreted in their youth versus how much mass they got through some other mechanism. For the first time with WFIRST, we'll be able to do the imaging tricks we do nowadays with the Gemini Planet Imager and Magellan, but do it on mature planets—no longer the adolescents. Middle-aged planets are much, much, much, much, much more common than adolescents, so we'll have a bigger sample to look at. We will actually see something that looks like a Jupiter and see a solar system that is analogous to our own. We'll see things about these planets' formation histories through their compositions that will serve a nice compliment to studies of younger planets.
Follette: As Bruce said, we're moving in a direction that I think is very promising. Looking even further ahead, NASA is studying four large, space-telescope mission concepts right now that might fly in the 2030s. Three of them would be very good candidates for doing direct imaging of exoplanets and/or exoplanet-forming disks. I think there's a real push in the field for one of the next big space missions to include a direct imaging component in its design, which will help us push toward imaging ever lower mass planets and better understand how planets evolve .
Macintosh: In the meantime, a lot of very powerful observations are going to be made by facilities like ALMA, the Atacama Large Millimeter/submillimeter Array, which is now up and running, and the James Webb Space Telescope when it flies later this decade. And when we get the next generation of extremely large, ground-based telescopes with 25- to 40-meter diameter primary mirrors in the 2020s, then, observationally, we really will get right into where planets form at Jupiter-like distances. We'll still have trouble pushing out to where Saturn and Uranus are located. But the next telescope wave will really let us see the equivalent of the inner Solar System caught in the process of forming.
Murray-Clay: This will be very important. The Kepler space telescope brought us thousands of planets, but in many cases we're only seeing one, easily detectable planet out of an entire solar system. By understanding the overall architecture of planetary systems in the future, we'll have a much better sense of the overall formation scenario for different kinds of systems.
Macintosh: A lot of us are driven by questions about Earth-like planets, of course. How common they are? How detectable might they be? Can we see them? Planet formation theorists like Ruth are trying to understand whether planets like Earth ultimately are a common thing or a rare thing, and whether our Solar System is unique or whether there's a whole bunch of others out there like it.
Follette: Never in the history of astronomy have we — meaning our star, our galaxy, and so on — proven to be particularly special, so it's not likely that our Solar System and its planets are one-of-a-kind. I find this typicality encouraging, because it means there's a chance that we'll image another Earth-like planet during my lifetime. To me, it ultimately means that we're not likely to be alone in the universe either.
Follow all of the Expert Voices issues and debates — and become part of the discussion — on Facebook, Twitter and Google+. The views expressed are those of the author and do not necessarily reflect the views of the publisher. This version of the article was originally published on Space.com.
Join our Space Forums to keep talking space on the latest missions, night sky and more! And if you have a news tip, correction or comment, let us know at: community@space.com.
Adam Hadhazy is a contributing writer for Live Science and Space.com. He often writes about physics, psychology, animal behavior and story topics in general that explore the blurring line between today's science fiction and tomorrow's science fact. Adam has a Master of Arts degree from the Arthur L. Carter Journalism Institute at New York University and a Bachelor of Arts degree from Boston College. When not squeezing in reruns of Star Trek, Adam likes hurling a Frisbee or dining on spicy food. You can check out more of his work at www.adamhadhazy.com.