A new approach to directly testing quantum gravity
Scientists have been trying to come up with a theory of quantum gravity for 100 years.
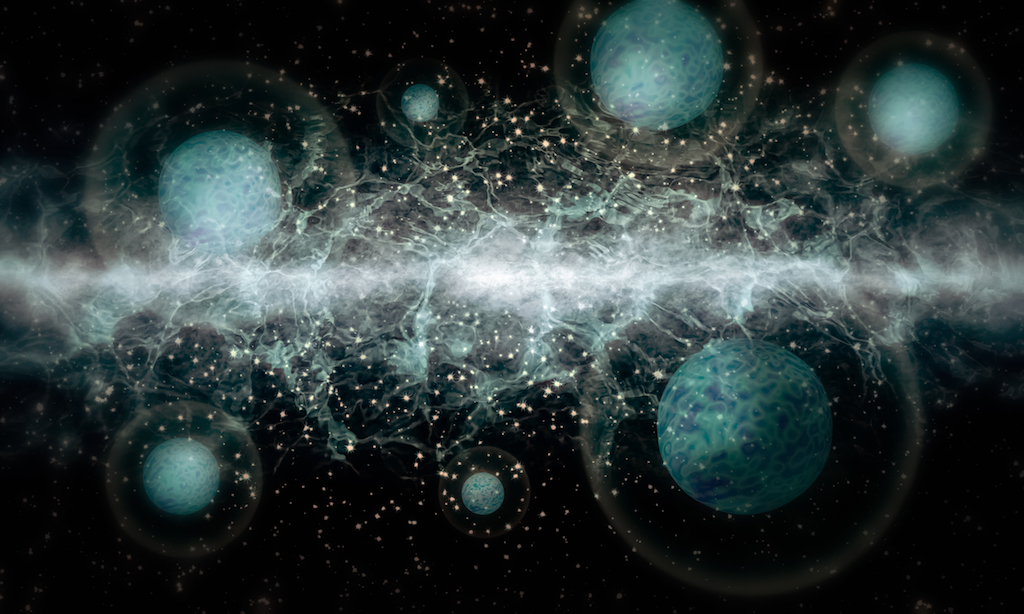
Paul M. Sutter is an astrophysicist at SUNY Stony Brook and the Flatiron Institute, host of Ask a Spaceman and Space Radio, and author of How to Die in Space. He contributed this article to Space.com's Expert Voices: Opinions and Insights.
It's the ultimate dream of modern physics, to achieve what generations of scientists have failed to do for 100 years: concoct a quantum theory of gravity.
But any theory will have to face observational tests, and observational tests of quantum gravity are hard to come by, because of the incredible energies needed. But a team of physicists has proposed a clever solution: refine an age-old technique, and use it to probe the tiniest scales in the universe.
The universe: Big Bang to now in 10 easy steps
Putting a spin on it
Back in 1922, in the glory days of expanding our view of the quantum world, Otto Stern and Walther Gerlach reported an exciting new discovery that rocked the foundations of physics. It was a pretty simple setup: shoot some atoms with an overall electric charge (say, silver ions, but pretty much anything will do) down a tube. Surrounding the tube is a particular configuration of magnets so that the magnetic field in the tube isn't perfectly even — it's stronger on the bottom than it is on the top.
If we pretend for a moment that atoms are tiny little balls of spinning electric charge, then those spinning charges will interact with the magnetic field. Depending on the direction that their spins point in, the thin beam of atoms should spray out in all sorts of directions. This is basic electrodynamics.
But they don't. Instead, the silver ions split into two uniform groups. One group is deflected up, and the other is deflected down. You could still think of atoms as tiny little balls of spinning electric charge, but that spin doesn't point in any random direction. According to the Stern-Gerlach experiment, there are only two possible directions for the spin to point in: all the way up or all the way down.
Get the Space.com Newsletter
Breaking space news, the latest updates on rocket launches, skywatching events and more!
This was the world's introduction to quantum spin, which is now a cornerstone of our understanding of fundamental particles. It also altogether accelerated our understanding of the quantum world: Without a full quantum understanding of the subatomic world, you couldn't explain the results of the Stern-Gerlach experiment.
Crossing path
And a full quantum understanding of the subatomic world is pretty bonkers. When you shoot the silver ions down the tube, they're not exactly sure which spin they're going to have — whether they will point up or down. Instead, in the language of quantum mechanics, they're said to exist in a superposition of states until they're observed (i.e., hit the wall at the end of the tube). The silver ions are both spin-up and spin-down, until they make a "choice" when the experiment is conducted.
It sounds crazy, but the math holds up. Using this language of superposition and probabilities, physicists are able to make predictions about how the subatomic world behaves.
Adding to the weirdness is the wave property of matter. It turns out that you can use the equations that govern wave motion to describe how those probabilities behave. In other words, the mathematics originally developed to figure out how ocean waves slosh back and forth was perfectly suited to describing how a subatomic particle would "decide" between being spin-up or spin-down.
No, it doesn't make any sense. And yes, it totally works.
We can take advantage of this wave property of matter to explore the quantum world even more deeply. For example, waves can interfere with each other, either amplifying (like that horrible feedback sound when you bring a microphone too close to a speaker) or canceling each other out (like the noise-canceling headphones you use to get some much-needed shuteye on intercontinental flights).
I used sound waves in my examples, but you could just as easily interfere water waves, light wave — and matter waves.
Researchers in the 1960s realized that they could use a Stern-Gerlach setup to build a system where the quantum waves of spin could interfere with each other, allowing for ultra-precise measurements. But the setup was deemed impractical — you would need exceedingly exquisite control over the magnetic fields in order to make the setup work.
Related: What is quantum gravity?
Into the quantum realm
But it's not the 1960s anymore, and a team of physicists have been able to make quantum waves of spin interfere with each other, as detailed in a paper recently appearing on the preprint journal arXiv.
It works like this. First you take some atoms and super-cool them. This makes them easier to handle. Then you send them into an atom chip, which is an experimental device to trap and control the flow of atoms. You then subject them to a magnetic field, where the wave nature of their quantum spin comes out — the atoms are now in a superposition of spin states. You split the beam of atoms, so that different atoms follow different paths, then you recombine them.
Depending on how you tune the lengths of the two paths, you can get either constructive or destructive interference. Because we're dealing with spins, either you get a whole lot of one spin or the other, or an even mix where everything washes out.
This setup lets you measure extremely tiny differences. If anything changes the length of the paths, or something messes with the spin nature of the atoms, then you can watch the resulting interference unfold.
The physicists haven't conducted any experiments with this device yet — they've spent years just making the thing work. But now that it does work, they can investigate a variety of physical processes, and possibly open the door to quantum gravity.
We don't have a fully quantum theory of gravity, which is needed to explain strong gravity at small scales. But this kind of spin interferometer can help us, because it's an easy-to-build device that directly probes the quantum world. For example, you could place a massive object (relatively speaking) next to one of the paths, and use the interference result to measure the strength of gravity at extremely tiny scales. As another example, the spin interferometer could be used as a tiny gravitational wave detector (as ripples in space-time would alter the lengths of the paths), operating at much different frequencies than the current generation of detectors.
Whatever's going on in the quantum world, the Stern-Gerlach experiment was one of the first to make it accessible. This device, developed almost a century later, could be the key to digging even deeper.
Read more: "Realization of a complete Stern-Gerlach interferometer: Towards a test of quantum gravity"
Follow us on Twitter @Spacedotcom or Facebook.
Join our Space Forums to keep talking space on the latest missions, night sky and more! And if you have a news tip, correction or comment, let us know at: community@space.com.
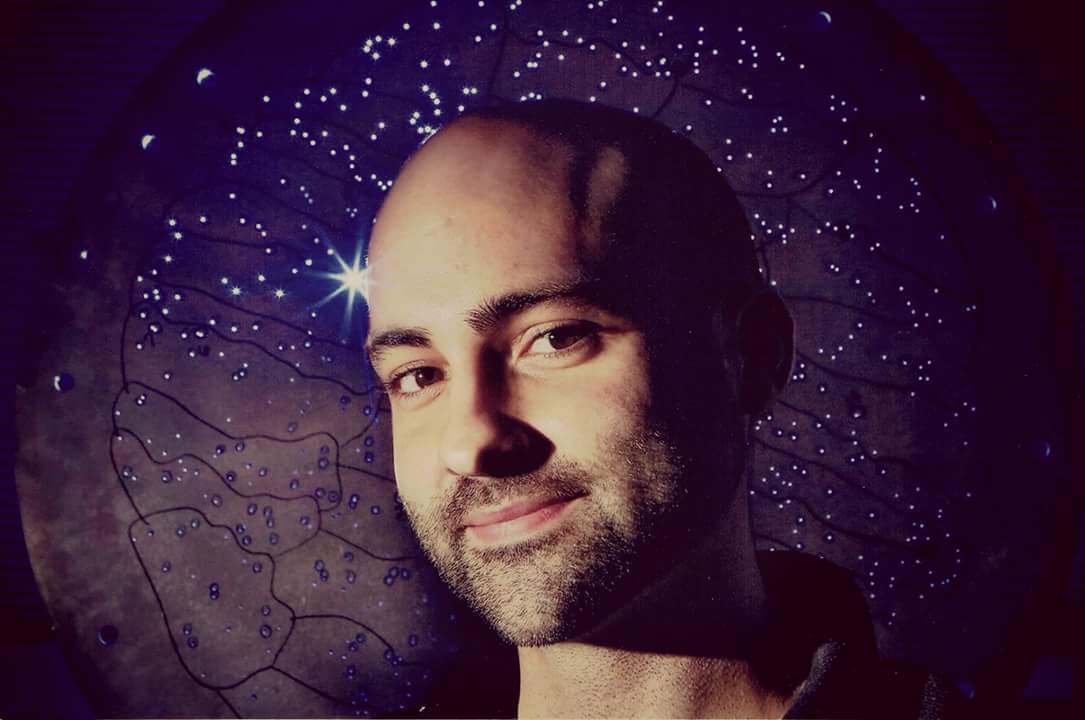
Paul M. Sutter is a cosmologist at Johns Hopkins University, host of Ask a Spaceman, and author of How to Die in Space.