James Webb Space Telescope finds neutron star mergers forge gold in the cosmos: 'It was thrilling'
"This is the first time we've been able to verify that metals heavier than iron and silver were freshly made in front of us."
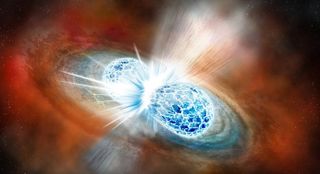
Scientists have analyzed an unusually long blast of high-energy radiation, known as a gamma-ray burst (GRB), and determined that it originated from the collision of two ultradense neutron stars. And, importantly, this result helped the team observe a flash of light emanating from the same event that confirms these mergers are the sites that create elements like gold.
The observations, made using the James Webb Space Telescope (JWST) and the Hubble Space Telescope, allowed scientists to see gold and heavy elements forged, which could help us better understand how these powerful neutron star merger events generate the only environments in the universe turbulent enough to create elements heavier than iron, such as silver and gold which results in a flash of light called a kilonova.
"It was thrilling to study a kilonova as we had never seen before using the powerful eyes of Hubble and JWST," research team member and University of Rome astrophysicist Eleonora Troja told Space.com. "This is the first time we've been able to verify that metals heavier than iron and silver were freshly made in front of us,"
Related: Dead star 'glitches' could reveal the origins of fast radio bursts
GRBs, which are the most powerful explosions of energy in the known universe, have been associated with neutron star mergers before — but this discovery is different.
These phenomena can be divided into two groups. On one hand, there are the long GRBs that last over 2 seconds and, on the other, short GRBs that last less than 2 seconds. While neutron star mergers have been associated with short GRBs, long GRBs were believed to occur as the result of the collapse of massive stars and not from such collisions.
The extremely bright and long burst, designated GRB 230307A, and detected by devices onboard NASA's Fermi mission in March 2023 lasted 200 seconds; this marked the second most energetic GRB ever seen. It seemed to be associated with a kilonova, designated AT2017gfo, and a neutron star merger that happened some 8.3 million light-years away, breaking the usual GRB convention and challenging theories of how these blasts of high-energy radiation are launched.
Get the Space.com Newsletter
Breaking space news, the latest updates on rocket launches, skywatching events and more!
"It is challenging to conceive that the duration of GRBs originating from compact binary mergers can extend to tens of seconds," Yu-Han Yang, research team leader and University of Rome postdoctoral astrophysicist, told Space.com.
Gamma-ray discovery could be a cosmic gold-mine
Stars are like stellar furnaces that forge the elements in the periodic table, beginning with the nuclear fusion of hydrogen to helium in their cores and continuing with the fusion of helium to heavier elements like nitrogen, oxygen and carbon.
The most massive stars, around 7 to 8 times as massive as the sun, can forge elements all the way up to iron in their hearts. Once a stellar core is filled with this element, fusion ceases. That also cuts off the outward energy line that had been supporting the star against its own gravity for millions, or sometimes billions, of years. The cores of these massive stars then collapse under this crushing gravity, blowing away their outer layers in supernova explosions.
This collapse transforms the stellar core, crushing electrons and protons into a sea of flowing neutrons, particles found in atomic nuclei that very rarely exist "freely." Yet, in this sea, the neutrons are prevented from squeezing close together by a quantum principle called neutron degeneracy pressure, which can be overcome with enough mass to create a black hole. But sometime there isn't enough mass for a black hole to come into existence.
Those dead stellar cores without the mass to overcome degeneracy pressure are left as 12-mile (20-kilometer) wide bosies with masses between one and two times that of the sun. However, there is a way that neutron stars can contribute heavier elements than iron to the universe.
Not all neutron stars exist alone.
Some traverse the cosmos in neutron star binary systems, meaning they have another neutron star in its gravitational clutches. As these dead stars orbit each other, they set the fabric of space ringing with ripples called gravitational waves that gradually carry away angular momentum from the system.
This causes neutron stars to spiral together, emitting gravitational waves faster as time passes and "leaking" more angular momentum in tandem. Ultimately, the two collide and merge. This collision creates a gamma-ray burst and sends out a spray of neutron-rich material that help create the heavier elements of the periodic table.
Other atomic nuclei around these collisions grab the free neutrons via the rapid-neutron capture process, or r-process, and become briefly lived superheavy elements called "lanthanides." Those lanthanides then quickly decay into lighter elements (though elements still heavier than lead.) This decay causes the emission of radiation, light we see from Earth as a "kilonova." Thus, tracking the evolution of kilonovas can help follow the creation of elements like gold and silver.
"Neutron star mergers could give rise to an ideal environment to extensively synthesize heavy elements, which is currently beyond artificial creation," Yang said. "Studying neutron star mergers helps us rewrite the obscure chapters of nucleosynthesis."
Cosmic alchemy in action
Over the course of weeks to months, Yang explained that kilonovas span a wide range of behaviors. These behaviors depend on the composition of the ejected material and the type of remnant formed at the center of the merger site.
Observations of most kilonovas do not extend to such late times in their evolution — but AT2017gfo was different. Unfortunately, however, the late-time observational data for AT2017gfo, collected with the Spitzer Space Telescope, were limited. They only offered weak signals contaminated by the kilonova's host galaxy and presented inadequate coverage in different wavelengths of light.
"During the first few days, the behavior of a kilonova is not affected by its chemical composition," Troja explained. "It takes weeks to reveal which metals are forged in the explosion, and we never had the chance to stare at a kilonova for that long."
These constraints had hindered scientists aiming to understand better kilonovas and the processes that create them.
In the case of AT2017gfo, however, the sensitivity and multi-color coverage of the JWST and Hubble observations allowed Yang and colleagues to observe the luminosity of this kilonova at late times.
"We tracked the evolution of the transient event associated with GRB 230307A up to two months after the burst and captured the full blue-to-red evolution of this transient, which can be classified as a kilonova," Yang said. "We discovered the recession of the photospheric radius at late times. The receding photospheric radius provides evidence for the recombination of heavy elements, such as lanthanides, occurring in the cooling process. Heavy r-process elements are needed to produce the observed data."
This confirmed that neutron star mergers do forge elements heavier than gold, and even confirms that long-GRBs can come from neutron star mergers. It hasn't, thought, solved the mystery of why this particular neutron star merger launched such an unusually long GRB.
"This event proves that a long-duration GRB coming from compact binary mergers is not a fortuitous occurrence," Yang said, adding there are lots of questions to still answer about these events. "What enlightening revelations can late-time observations of kilonovas offer on nucleosynthesis?
"We look forward to joint observations of long-duration gamma-ray bursts, kilonovas and gravitational waves in the future, which will help unveil the mysteries about such outliers."
The team's research was published on Wednesday (Feb. 21) in the journal Nature.
Join our Space Forums to keep talking space on the latest missions, night sky and more! And if you have a news tip, correction or comment, let us know at: community@space.com.
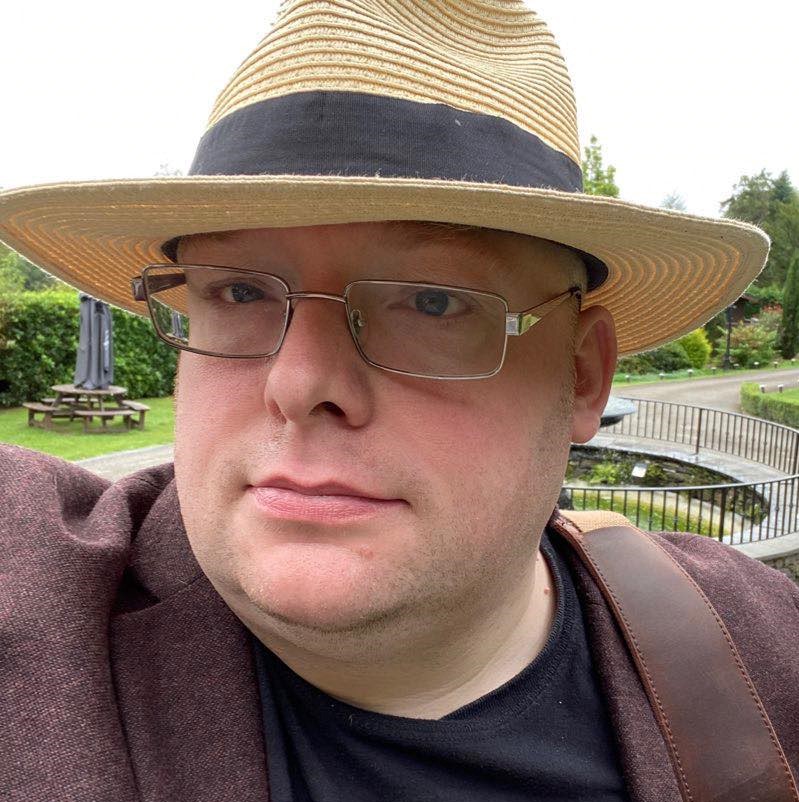
Robert Lea is a science journalist in the U.K. whose articles have been published in Physics World, New Scientist, Astronomy Magazine, All About Space, Newsweek and ZME Science. He also writes about science communication for Elsevier and the European Journal of Physics. Rob holds a bachelor of science degree in physics and astronomy from the U.K.’s Open University. Follow him on Twitter @sciencef1rst.