Higgs boson: The 'God Particle' explained
The existence of the Higgs boson completes the standard model of particle physics.
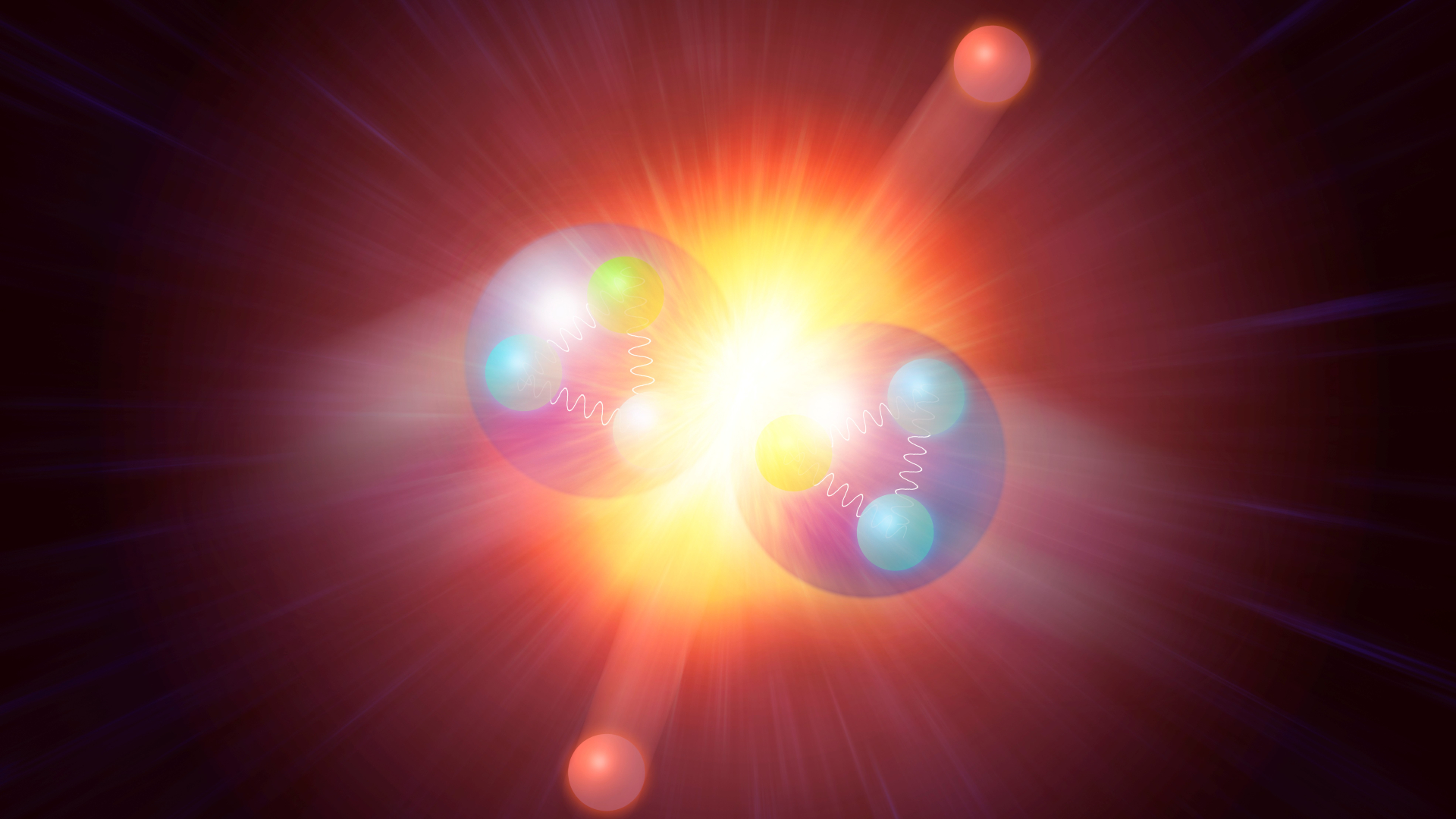
On April 8, 2024, British theoretical physicist Peter Ware Higgs, who first theorized the existence of the Higgs Boson, passed away at the age of 94. His passing was announced on April 10.
"Besides his outstanding contributions to particle physics, Peter was a very special person, an immensely inspiring figure for physicists across the world, a man of rare modesty, a great teacher, and someone who explained physics in a very simple and yet profound way," Fabiola Gianotti, CERN Director General, said on the institution's X feed. "An important piece of CERN’s history and accomplishments is linked to him. I am very saddened, and I will miss him sorely."
The Higgs boson is the fundamental force-carrying particle of the Higgs field, which is responsible for granting other particles their mass. This field was first proposed in the mid-sixties by Peter Higgs — for whom the particle is named and his colleaguees.
The particle was finally discovered on July 4, 2012, by researchers at the Large Hadron Collider (LHC) — the most powerful particle accelerator in the world — located at the European particle physics laboratory CERN, Switzerland.
The LHC confirmed the existence of the Higgs field and the mechanism that gives rise to mass and thus completed the standard model of particle physics — the best description we have of the subatomic world.
Related: The Higgs boson could have kept our universe from collapsing
As scientists approached the end of the 20th Century advances in particle physics had answered many questions that surrounded the fundamental building blocks of nature. Yet, as physicists steadily populated the particle zoo with electrons, protons, bosons, and all flavors of quarks, some pressing questions remained stubbornly unanswered. Amongst these, why do some particles have mass?
The story of the Higgs boson is motivated by this question.
What is the Higgs boson?
The Higgs boson has a mass of 125 billion electron volts — meaning it is 130 times more massive than a proton , according to CERN. It is also chargeless with zero spin — a quantum mechanical equivalent to angular momentum. The Higgs Boson is the only elementary particle with no spin.
A boson is a "force carrier" particle that comes into play when particles interact with each other, with a boson exchanged during this interaction. For example, when two electrons interact they exchange a photon — the force-carrying particle of electromagnetic fields.
Because quantum field theory describes the microscopic world and the quantum fields that fill the universe with wave mechanics, a boson can also be described as a wave in a field.
So a photon is a particle and a wave that arises from an excited electromagnetic field and the Higgs boson is the particle or "quantized manifestation" that arises from the Higgs field when excited. That field generates mass via its interaction with other particles and the mechanism carried by the Higgs boson called the Brout-Englert-Higgs mechanism.
Why is the Higgs boson called the 'God Particle?'
The Higgs boson's nickname "the God Particle" was solidified upon its discovery, namely as a result of the popular media. The origin of this is often connected to Nobel Prize-winning physicist Leon Lederman referring to the Higgs boson as the "Goddamn Particle" in frustration with regards to how difficult it was to detect.
Business Insider says that when Lederman authored a book on the Higgs boson in the 1990s the title was to be "The Goddamn Particle" but the publishers changed this to "The God Particle" and a troublesome connection with religion was drawn, one which bothers physicists to this day.
Still, it's hard to overestimate the importance of the Higgs boson and the Higgs field in general, as without this aspect of nature no particles would have mass. That means no stars, no planets, and no us — something which may help warrant its hyperbolic nickname.
Why is the Higgs boson important?
In 1964, researchers had begun to use quantum field theory to study the weak nuclear force — which determines the atomic decay of elements by transforming protons to neutrons — and its force carriers the W and Z bosons.
The weak force carriers should be massless, and if they weren't this risked breaking a principle of nature called symmetry which — just like the symmetry of a shape ensures it looks the same if it is turned or flipped — ensures the laws of nature are the same however they are viewed. Putting mass arbitrarily onto particles also caused certain predictions to trend towards infinity.
Yet, researchers knew that because the weak force is so strong over short distance interactions — much more powerful than gravity — but very weak over longer interactions, its bosons must have mass.
The solution proposed by Peter Higgs François Englert, and Robert Brout, in 1964 was a new field and a way to "trick" nature into breaking symmetry spontaneously.
An article from CERN compares this to a pencil standing on its tip — a symmetrical system — suddenly tipping to point in a preferred direction destroying its symmetry. Higgs and his fellow physicist proposed that when the universe was born it was filled with the Higgs field in a symmetrical, but unstable state — like the precariously balanced pencil.
The field quickly, in just fractions of a second, finds a stable configuration, but this in the process breaks its symmetry. This gives rise to the Brout-Englert-Higgs mechanism which grants mass to the W and Z bosons.
What was later discovered about the Higgs field was that it would not only give mass to the W and Z bosons but that it would grant mass to many other fundamental particles. Without the Higgs field and the Brout-Englert-Higgs mechanism, all fundamental particles would race around the universe at the speed of light. This theory doesn't just explain why particles have mass but also, why they have different masses.
Particles that interact — or "couple" — with the Higgs field more strongly are granted greater masses. Even the Higgs boson itself gets its mass from its own interaction with the Higgs field. This has been confirmed by watching how Higgs boson particles decay.
One particle not granted mass by the Higgs field is the basic particle of light — the photon. This is because spontaneous symmetry breaking doesn't happen for photons as it does for its fellow force-carrying particles the W and Z bosons.
This mass-granting phenomenon also only applies to fundamental particles like electrons and quarks. Particles like protons — made up of quarks — get most of their mass from the binding energy that holds their constituents together.
While all this conforms well to theory, the next step was to discover evidence of the Higgs field by detecting its force-carrying particle. Doing this would be no simple task, in fact, it would require the largest experiment and most sophisticated machine in human history.
In this way, the search for the Higgs boson itself has pushed both particle accelerator and detector technology to its limits — with the ultimate expression of this being the Large Hadron Collider (LHC).
Higgs boson discovery and the standard model
Detecting the Higgs boson isn't just a question of setting up a detector and waiting for one to come along. These particles only existed in the high-energy conditions of the early universe.
That means before detecting this particle, these high-energy conditions have to be replicated and Higgs bosons have to be created. The LHC does this by accelerating protons to near-light speed and smashing them together.
This creates a cascade of particles that quickly decay into lighter particles. The Higgs boson decays too rapidly to be spotted and was instead identified by detecting particle decays that indicated a particle with no spin and matched theoretical predictions for this missing boson.
The particle was detected by both the LHC ATLAS detector and the Compact Muon Solenoid (CMS) detector.
The announcement of the detection of the Higgs boson was made at CERN in Geneva on July 4, 2012. It took until March the following year to confirm this the detected particle was indeed the Higgs boson.
By revealing this particle, predicted by the standard model the discovery of the Higgs boson completed this picture of the subatomic world. There are still mysteries beyond this theory such as the nature of dark matter which the Higgs boson — through its unique properties — could help solve.
The Higgs boson after 2012
The year after the discovery of the Higgs boson, Peter Higgs and François Englert were awarded the 2013 Nobel Prize in Physics, for their Higgs field theory.
The Nobel Committee wrote of the award: "for the theoretical discovery of a mechanism that contributes to our understanding of the origin of mass of subatomic particles, and which recently was confirmed through the discovery of the predicted fundamental particle, by the ATLAS and CMS experiments at CERN's Large Hadron Collider."
The discovery of the Higgs boson may have completed the standard model but this wasn't the end of the investigation of this elusive particle. One of the major discoveries made since 2012 has involved confirmation of the decay of the Higgs.
And the investigation of this elusive particle will deepen during run 3 of the LHC and particularly when the particle accelerator's high luminosity upgrade is completed in 2029.
This will allow the LHC to conduct more collisions providing researchers with more opportunities to spot exotic physics including phenomena beyond the standard model.
CERN estimates that following the upgrade each year the accelerator will create 15 million of these particles. This is compared to 3 million Higgs bosons created by the LHC in 2017. This may be key to detecting other "flavors" of the Higgs boson.
Theories that go beyond the standard model of particle physics also predict as many as five different types of Higgs bosons which may be produced more infrequently than the primary Higgs boson. Even before the upgrades, scientists have already provided us with tantalizing evidence of a "magnetic Higgs boson."
Higgs boson expert Q&A
We spoke to Yves Sirois, physicist and research director at École Polytechnique about the Higgs Boson.
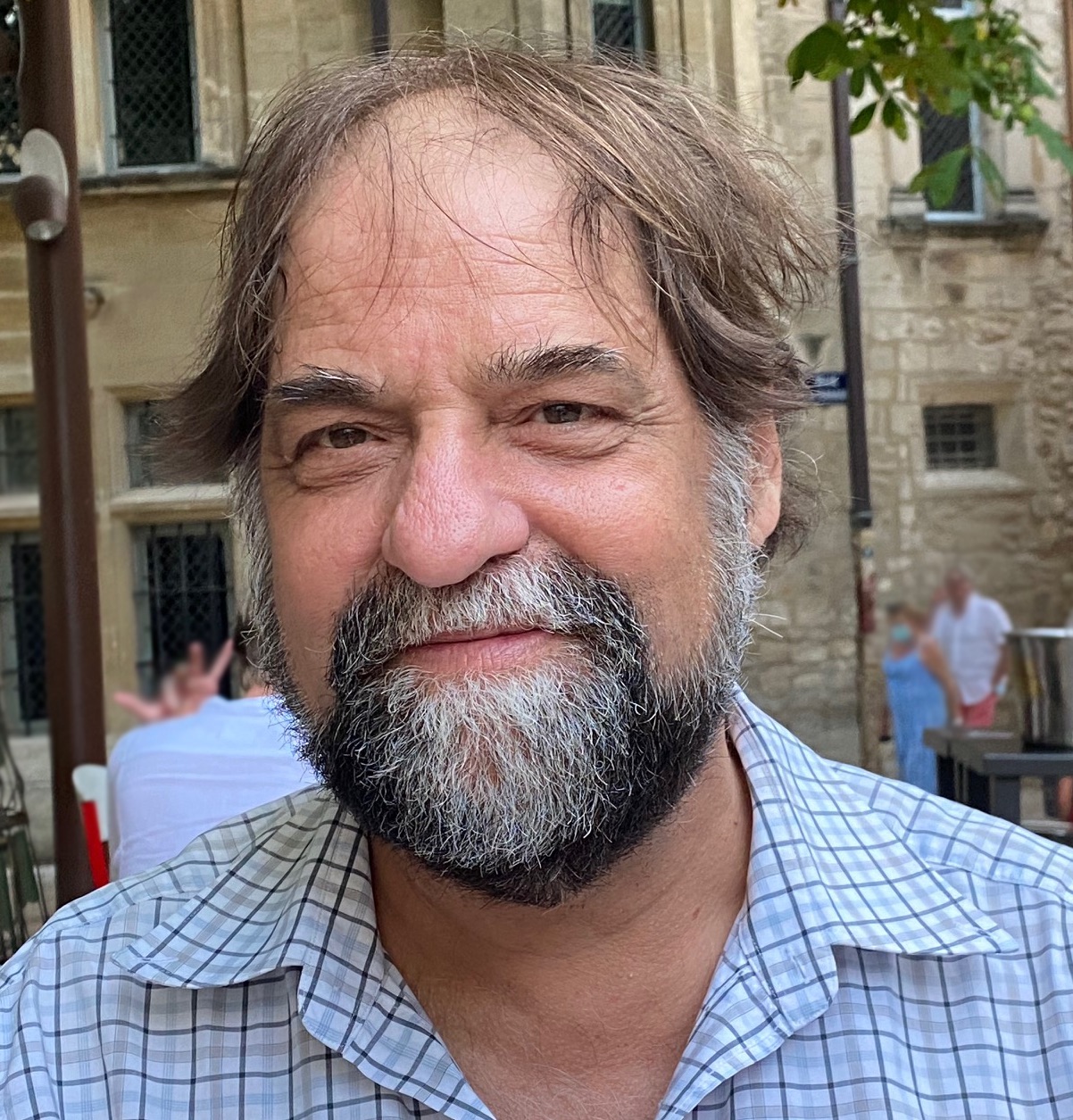
Yves Sirois is a physicist on the CMS experiment at CERN and has been director of the Leprince-Ringuet Laboratory at Institut Polytechnique de Paris since January 2020.
What is the Higgs Boson?
The Higgs boson is an elementary particle associated with the Higgs field. It is the quantum excitation of this field, like ripples on the sea. The boson itself is a completely new kind of animal in the zoo of particles. It has neither the quantum properties of elementary matter nor those of the carriers of quantum interactions such as the electromagnetic force, weak force, or nuclear interactions.
Who first theorized about the Higgs Boson and why?
The existence of the Higgs boson was theorized in the mid-1960s as a consequence of a symmetry-breaking mechanism suggested by Peter Higgs, François Englert, and Robert Brout, during the summer of 1964, and also independently in the early fall of the same year by a U.S./U.K. group composed of Gerald Guralnik, Carl Hagen, and Tom Kibble.
Without this mechanism, it seemed impossible to construct a theory of the fundamental interactions that would be valid at all energy scales. In particular, one could not explain the existence of the weak interaction that only acts at very short distances, and is responsible for radioactivity within the nucleus of an atom.
Why is it such an important particle?
This Higgs field played an absolutely decisive role in the first moments after the birth of the universe as it determines the very nature of the vacuum which fills our space-time. It makes the existence of matter and interactions as we know them possible, and it is responsible for the appearance of the mass of all known elementary particles. Without the Higgs field, and thus without the Higgs boson, there would just be no atomic elements, no stars, and no life in this universe.
How was the Higgs Boson discovered?
The Higgs boson was discovered at the Large Hadron Collider (LHC) simultaneously by the two large multipurpose experiments ATLAS and CMS. The discovery was announced on the 4th of July 2012 at CERN.
The new scalar (spin 0) boson was observed at the time mainly in two rare decay channels offering the cleanest signal, the decay in a pair of photons, which involved a purely quantum virtual process, and the direct decay in a pair of Z bosons, the carrier of the neutral weak interaction.
Why did the search for this particle take so long?
This is because the Higgs boson is both very heavy and utterly unstable!
With a mass of 125 GeV, it is 133 times heavier than a hydrogen atom. Its mean lifetime is between one and two ten-thousandth of a billionth of a billionth of a second (about 1,6 x 10^-22 s).
To produce such a heavy particle, given the familiar E = mc^2 equation, you basically have to concentrate a considerable amount of energy in a small volume. This is why we needed a high-energy collider. The LHC at CERN is the most powerful such machine existing on Earth. The Higgs boson, ounce produced, will decay in many different ways, and only a small fraction of these can be distinguished from the common background. To detect and identify such particles, we have constructed the largest and most complex detectors ever conceived. We then analyzed hundreds of billions of proton-proton collision events to extract a Higgs boson signal.
Why do scientists such as yourself still investigate the Higgs Boson?
For two main reasons besides the obvious necessity to perform the most precise characterization of the new particle. Firstly, we want to understand how the physical vacuum in which we live was created in the early universe. Secondly, we want to understand which this physical vacuum can be made stable.
The Higgs field is very unique as it provides a non-zero mean expected potential energy to the physical vacuum! This is, fundamentally, what makes the whole difference for our universe. We believe that it is possible to access the shape of the energy potential which is responsible for the electroweak symmetry breaking, that is of the very existence of the electromagnetic force of infinite range and of the weak force acting at very short ranges. It turns out that to do this, we have to study the way the Higgs bosons interact with themselves! And for this, we need to produce pairs of Higgs bosons!
The Higgs boson solved the question of the origin of the mass of all other elementary particles, but its own mass is unexplained. This mass is not protected by any symmetry of the theory, and it brings unwanted instability in the theory. So much, so that via quantum processes, the physical vacuum itself could become destabilized by quantum fluctuations. Fortunately, this is on timescales considerably larger than the known lifetime of our universe, but we are nevertheless looking for additional Higgs boson-like particles that would signal the existence of the new physics needed to stabilize the Higgs boson mass.
All this motivates the analysis of much more data and, if possible, access to higher collider energies. This is why thousands of scientists from all around the world are still dedicated to the task!
Additional Reading
The discovery of the Higgs boson completed what is known as the standard model of particle physics. CERN explains what this framework tells us about the subatomic world. Learn more about the Higgs boson with this article from the U.S. Department of Energy. Explore some frequently asked questions about the Higgs boson with CERN.
Bibliography
The Higgs Boson, CERN, https://home.cern/science/physics/higgs-boson
The Higgs Boson, the Department of Energy, https://www.energy.gov/science/doe-explainsthe-higgs-boson
What's so special about the Higgs boson?, CERN, https://home.cern/science/physics/higgs-boson/what
Higgs. P., BROKEN SYMMETRIES AND THE MASSES OF GAUGE BOSONS, Physical Review Letters, [1964], [https://journals.aps.org/prl/pdf/10.1103/PhysRevLett.13.508]
Peter W. Higgs, The Nobel Prize, https://www.nobelprize.org/prizes/physics/2013/higgs/facts/
High-Luminosity LHC, CERN, https://home.cern/science/accelerators/high-luminosity-lhc
Join our Space Forums to keep talking space on the latest missions, night sky and more! And if you have a news tip, correction or comment, let us know at: community@space.com.
Breaking space news, the latest updates on rocket launches, skywatching events and more!
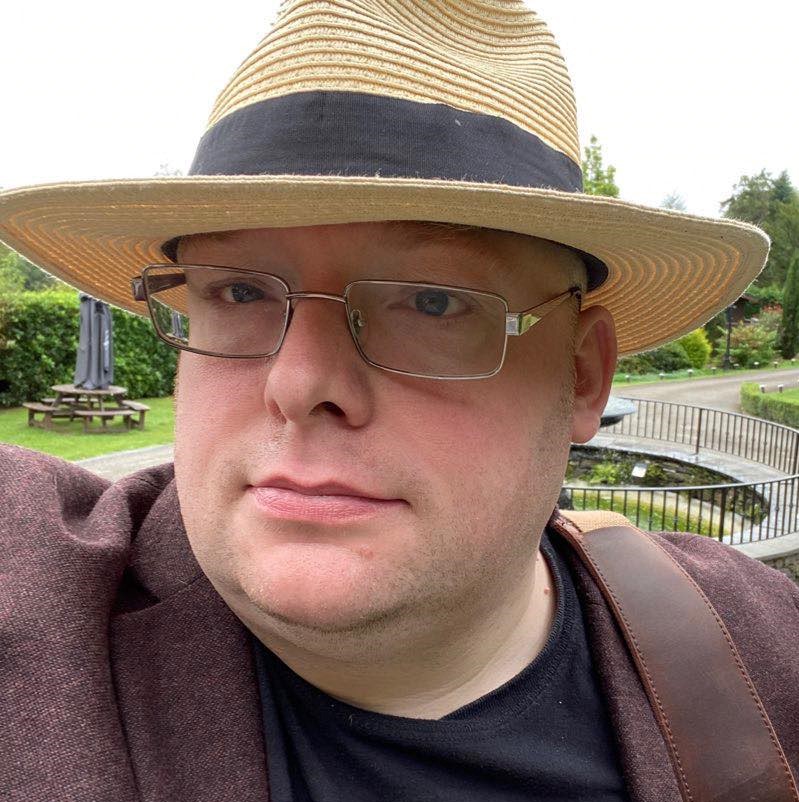
Robert Lea is a science journalist in the U.K. whose articles have been published in Physics World, New Scientist, Astronomy Magazine, All About Space, Newsweek and ZME Science. He also writes about science communication for Elsevier and the European Journal of Physics. Rob holds a bachelor of science degree in physics and astronomy from the U.K.’s Open University. Follow him on Twitter @sciencef1rst.