A cosmic magnifying glass: What is gravitational lensing?
Here we explore how gravitational lensing is helping scientists see the invisible.
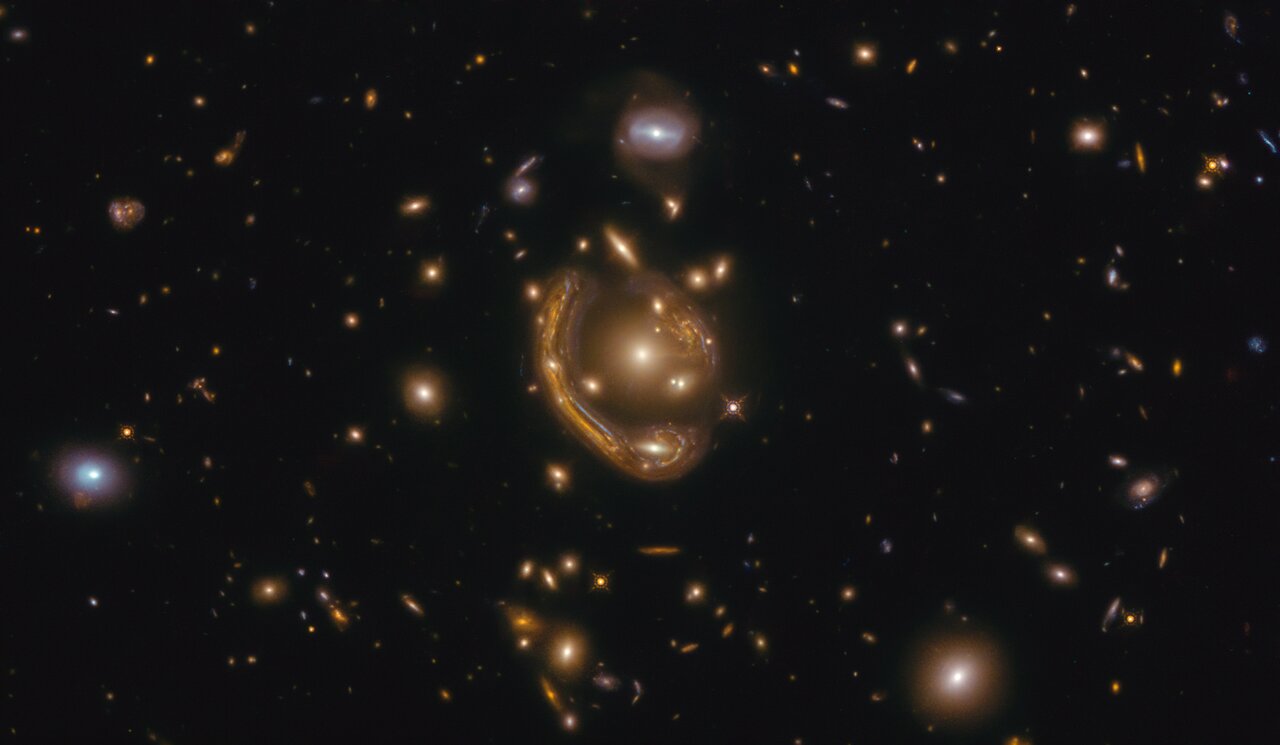
Gravitational lensing is an effect on light from a background source that arises as a result of the curvature of spacetime, the three dimensions of space and time united into a single entity, caused by mass.
The effect is most observable when light from a bright background source, like a star, a quasar, or an entire galaxy, passes a very massive object like another galaxy or a cluster of galaxies, described as a lensing object or just a gravitational lens.
This can have several results; it can make an object shift its apparent position in the sky over Earth, or it can cause a single object to appear at multiple points in the sky, occasionally giving rise to spectacular formations like rings and crosses made from the same object.
More than this, gravitational lensing can actually cause the light from a background object to be amplified. That means that astronomers can use the gravitational lensing arising from galactic clusters as natural cosmic magnifying glasses.
This has made it an important tool for the investigation of the universe when it was in its infancy, making light from the earliest galaxies that would usually be too faint to see observable by instruments like the James Webb Space Telescope and the Hubble Space Telescope, NASA says.
Related: James Webb Space Telescope image gallery
How does gravitational lensing work?
In 1915 Albert Einstein revolutionized how we think of gravity by introducing general relativity, a theory that is also sometimes known as the geometric theory of gravity. It is from this theory that gravitational lensing was born.
Einstein's idea was that gravity arises from the fact that mass causes the very fabric of spacetime to curve, and the greater the mass, the greater this curvature is. Think of this as being analogous to balls of increasing mass being placed on a stretched rubber sheet, with a bowling ball causing a greater "dent" than, say, a tennis ball.
Of course, this curvature has an effect on other matter passing over it. So, for instance, the curvature of spacetime caused by the sun keeps Earth in orbit, while the curvature Earth itself causes keeps the moon in orbit [R. J. A Lambourne., 2010, pg 166].
American theoretical physicist John Wheeler succinctly described the effect of general relativity as such: "Matter tells space-time how to curve, and space-time tells matter how to move."
But, the curvature of spacetime doesn't just affect matter; it affects light too, meaning that light always travels in straight lines, apart from when it doesn't. If this sounds contradictory, think of a straight line drawn on a sheet of paper. If that paper is then curved, the line itself hasn't deviated from its path, but yet it is still no longer straight. The path photons of light follow as they travel through space is called a geodesic, and it can be curved like a line drawn on paper [R. J. A Lambourne., 2010, pg 133].
The bending of light as it passes a curved region of spacetime created by a massive object gives rise to gravitational lensing [R. J. A Lambourne., 2010, pg 223].
Until general relativity, in the physics of Issac Newton, space and time had been considered the unchanging stages upon which the events of the universe played out, though Newton had also predicted the bending of light but to a much lesser extent than Einstein.
Changing spacetime into a dynamic and changing aspect of the universe was controversial and meant general relativity would require a great deal of evidence before it would be accepted by the physics community of the 20th century. Fortunately, gravitational lensing provided exactly the kind of predictable and observable effect that could be used to deliver this evidence.
How gravitational lensing proved Einstein right
General relativity suggests that as an upshot of gravitational lensing, the curvature of light from a background source as it passes a gravitational lens causes the object it originates from appear in a different location in the sky than it would normally.
Astronomer Arthur Stanley Eddington believed that this shift in apparent position was key to providing verification of general relativity. He thought that he could use a solar eclipse and the darkening of the sun to observe the apparent position shift of well-studied stars caused by the mass of the sun.
Eddington took advantage of the 1919 eclipse to test this idea, traveling to Sobral in northern Brazil to observe the eclipse while a second team journeyed to the island of Príncipe off the coast of West Africa to make similar observations.
During the 1919 eclipse, the sun sat in front of the Hyades, a cluster of bright stars in the constellation of Taurus. The light-bending effect would be at its most extreme closest to the disk of the sun, and fortunately, many stars of the Hyades would be visible near the disk of the sun during the eclipse.
Despite many technical issues experienced during the double expedition, Eddington and the second team led by astronomer Andrew Claude de la Cherois Crommelin observed a deflection of light from these stars as a result of the sun coming between them and Earth that was consistent with the predictions of general relativity. The change in apparent position was twice that which was predicted by Newton's theory of gravity.
Though the findings have not been without controversy, many similar eclipse experiments performed after this have further confirmed gravitational lensing and the curvature of space by massive objects and have revealed more about this incredible phenomenon arising from gravity.
Types of gravitational lensing
There are three major types of gravitational lensing, according to the University of California, Berkeley, strong lensing, weak lensing, and microlensing.
Strong gravitational lensing
As the name suggests, strong lensing is the most extreme of these and occurs when the gravitational lens is particularly massive, and the background source that is being lensed is close to it. This means that the light from this source can take multiple paths past the gravitational lens, depending on how close its path carries it. As a result, strongly lensed light from a single object can arrive at an observer at different times.
If the background object that is being lensed varies with time, then its multiple images will also vary. Not only can this be used to track the development of explosive events like supernovas, the explosive deaths of massive stars, but it can also be used to measure how rapidly the universe is expanding, a rate known as the Hubble constant, University of California, Berkeley explains.
Results of strong gravitational lensing
The first time that multiple images were seen from a single object was in 1979 when astronomers saw the double image of a quasar, which has come to be known, somewhat inaccurately, as the "Twin Quasar."
Initially believing these to be two separate quasars, designated Q0957+561 A and B, astronomers studied their radio and visible light spectra, discovering they are identical. A team of scientists led by Dennis Walsh concluded that these twin quasars are, in fact, the same object, the light from which has taken different paths around a faint but detectable galaxy between the quasar and Earth, with that galaxy acting as a gravitational lens [R. J. A Lambourne., 2010, pg 223].
Since 1979, astronomers have discovered that stang gravitational lensing can create some weird and wonderful manifestations.
According to ESA, the results of strong gravitational lensing are different depending on the shape of the object that is doing the lensing. The simplest types of gravitational lensing occur when there is a single object warping spacetime and bending light.
If a gravitational lens is spherical, then it creates what is known as an Einstein ring in which a single object is repeated in a circular arrangement. If the gravitational lens object is elongated, like some galaxies, for instance, that the background object is replicated in a cross-like arrangement, referred to as an Einstein cross.
ESA adds that more complex gravitational lensing happens when the lensing object is an irregular shape or an arrangement of massive objects, such as a galactic cluster. In these cases, the effect on background sources is warping their appearance, smearing them across an image, and making them appear as arcs or even stretched out like taffy.
This striking effect is particularly prominent in the arcs and swirls that represent lensed galaxies seen in the first image delivered to the public from the James Webb Space Telescope (JWST), the deep field image of galaxy cluster SMACS 0723 revealed by U.S. President Joe Biden on July 11, 2022.
These smears created by galactic clusters acting as gravitational lenses can be studied to assess the distribution of mass within those clusters. This is particularly useful to astronomers studying the distribution of dark matter around galaxies.
Though dark matter doesn't interact with electromagnetic radiation and thus doesn't emit, absorb, or reflect light, it does have mass, meaning it warps spacetime and interacts gravitationally, just like "ordinary matter" that makes up the visible components of galaxies.
That means by looking at the amount of gravitational lensing caused by a galaxy or a cluster of galaxies and then comparing this to the lensing that would have arisen from just the visible matter in that gathering, like stars and hot gas, astronomers can determine how much invisible dark matter is present and how it is distributed.
Weak and micro gravitational lensing
Weak lensing occurs when the gravitational lensing isn't extreme enough to give rise to multiple instances of the same object in the same view of the universe or to create visually striking smeared galaxies. Weak lensing still causes some distortion, but this can't be seen on individual galaxies, so the only way to really see the effect of weak lensing is by looking at a lot of galaxies and averaging the effect across them.
Strong and weak gravitational lensing come from incredibly massive objects like galaxies or galactic clusters, but much more diminutive objects can also warp spacetime and divert the path of light. Gravitational microlensing [R. J. A Lambourne., 2010, pg 225] occurs when a lensing object has a mass similar to that of the sun or as large as several times that of our star.
While the distortion created by gravitational microlensing may be too subtle to detect, it does create a brightening of objects. This means gravitational microlensing can be used by monitoring changes in the brightness of well-studied stars. The brightening of a distant star for a period of days or weeks can indicate that a dense and dark unseen object has passed in front of these stars, causing them to be temporarily lensed.
Microlensing has become a viable way to detect black holes, which don't emit any light from beyond the light-trapping surface that acts as their boundary, known as the event horizon, and thus can't be seen unless they are creating turbulent and violent conditions in gas and dust around them causing it to glow. This is because as they still possess mass, black holes still warp space and thus still give rise to a small amount of gravitational lensing.
How the JWST and Hubble space telescope use gravitational lensing to look back in time
As the light from distant and thus early galaxies travels to Earth, it loses energy and thus becomes fainter. That means early galaxies are so faint they aren't visible to even the most powerful equipment created by humanity. That is unless they get a helping handing from a magnifying glass the size of an entire galactic cluster.
The magnification of light caused by gravitational lensing has been used to great effect by the Hubble Space Telescope, which has employed it to study the structure of early galaxies. From its position over Earth, free of the blurring effects of our planet's atmosphere, Hubble, which has been studying the universe since 1990, can see gravitationally lensed early galaxies that ground-based telescopes would miss.
This has helped the ground-breaking telescope to study the structure of galaxies that could not be seen without the use of gravitational lensing, even by Hubble's new, more powerful partner, the James Webb Space Telescope (JWST) according to NASA.
The JWST has followed the lead of Hubble, using gravitational lensing to great effect and producing images with galaxies warped and smeared around a galactic cluster lens in such a way it would make abstract painter Salvador Dali proud.
In just its first year of operation since coming online in mid-2022, the JWST has built upon the work of Hubble using gravitational lensing to see four of the most distant and, thus, earliest galaxies known to date. These galaxies, JADES-GS-z10–0, JADES-GS-z11–0, JADES-GS-z12–0, and JADES-GS-z13–0, existed when the 13.8 billion-year-old universe was just around 350 million years old.
Gravitational lensing FAQs answered by an expert
We asked Victor Chan, a Ph.D. student in the University of Toronto's David A. Dunlap Department of Astronomy & Astrophysics, some frequently asked questions about gravitational lensing.
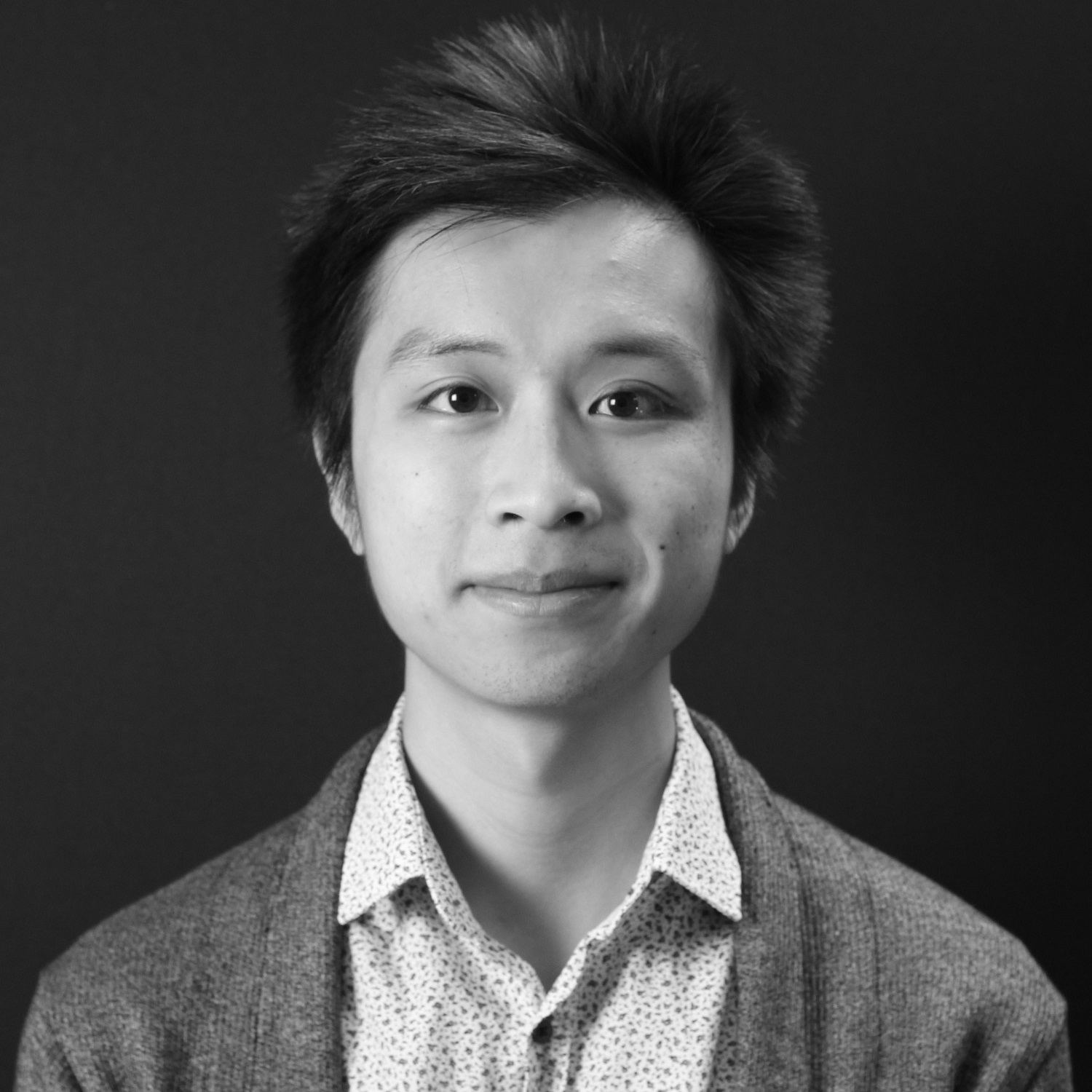
Victor Chan is a Ph.D. student in the University of Toronto's David A. Dunlap Department of Astronomy & Astrophysics. He specializes in cosmology, cosmic microwave background, data modeling and analysis.
What is gravitational lensing and what causes it?
Gravitational lensing is when light is deflected by objects with very strong gravity. We normally think of light traveling in straight lines. For example, you can see the fire on a candle because its light travels straight to your eyes. Sometimes the path that a light ray takes can be deflected, and we generally refer to this as lensing. We see this happen in everyday life when light travels from one medium into another medium with different density. This is how glasses work. Gravitational lensing also refers to the bending of a light path, but this time it's due to gravity! Just like how gravity can affect the path of regular objects, light rays can be deflected by objects with very large mass.
When does gravitational lensing occur?
Three things are required! First, we require an observer. This is usually someone, or a telescope, on Earth. Next, we need a lens. Again, a very large mass is needed to significantly change the path of light, so this is usually a galaxy or a galaxy cluster. Finally, we need a source of light that will be lensed. The geometry of gravitational lensing generally requires the source to be behind the lens from the perspective of the observer. This specific configuration means that it's relatively rare for us to observe gravitationally lensed objects.
How can astronomers use gravitational lensing?
It takes A LOT of mass to significantly deflect light away from its original path. It usually requires the mass of a galaxy or even a cluster of galaxies. This is why gravitational lensing is usually only observed at cosmic scales. Just like a magnifying glass, lensed light can be magnified or de-magnified. If the light coming towards us from a distant source is lensed by a massive galaxy located in between, then we can take advantage of magnified (and therefore brighter) images to study them in more detail. We can also learn about the mass of the lens itself by studying how strongly it lenses the light around it.
Why is it useful in seeing early galaxies?
Light speed is a finite quantity, so it takes time for light to travel cosmic distances. The "lookback time" of early galaxies tells us that the ones we can see are located very far away. This makes them difficult to observe because of their apparent size and brightness, and gravitationally lensed images of these faraway galaxies (specifically the magnified versions) can be better observed and studied since they are brighter than their un-lensed counterparts. I like to think of it as if we are putting those faraway galaxies under a microscope (of course, that's not what is actually happening, but the idea is similar).
How is gravitational lensing related to dark matter?
Dark matter is anything that interacts gravitationally (just like regular matter) but does not directly emit or absorb light. Astronomers know it exists because we see the effects of gravity at cosmic scales, and they observe stronger gravitational effects than visible matter can account for. The same thing applies to gravitational lensing. The extent to which images are magnified by gravitational lenses is much stronger than the visible matter in the lenses can account for. If we compare the mass of the gas and stars we see in a galaxy to the mass we infer from gravitational lensing, we tend to notice that there is a lot of mass that we do not see. We can then infer how much dark matter must be present in the lens in order for us to observe such a degree of lensing.
Are there different types of gravitational lensing
Yes! There are several kinds of gravitational lensing, but they can be described by the same phenomenon of light paths being deflected by gravity. Sometimes astronomers differentiate between strong and weak lensing, which refers to how much the path of light has been deflected (the angle between the original and deflected paths). Images can be magnified or demagnified, as I described earlier, but they can also be warped, which astronomers refer to as shear lensing). Astronomers also sometimes distinguish between the type of light that is being observed. Galaxy lensing is usually observed with optical light. We can also observe gravitational lensing with microwave light leftover from the Big Bang!
How do you use gravitational lensing in your research?
I study the effects of gravitational lensing on the cosmic background radiation (also called the cosmic microwave background or CMB) from the Big Bang. This is residual light leftover from when the Big Bang, and we can observe it from virtually every direction. In some sense, it is the perfect source of light to be gravitationally lensed because this light comes to us from behind all of the cosmic structures and galaxies that formed after the Big Bang. The problem is that we don't know how the original CMB looked since we can only observe the lensed version. My research focuses on taking advantage of our knowledge of how gravitational lensing works to disentangle the information about the Universe's massive structures in the observed CMB.
Additional resources
General relativity explains that the curving of spacetime by mass gives rise to other stunning and often shocking phenomena aside from gravitational lensing. One example is "frame dragging," in which a rotation object of great mass literally drags space and time around with it. In 2015 an astronomer from the University of California, Berkeley, discovered a distant supernova being lensed four times by a massive galaxy creating an explosive Einstein cross.
Bibliography
Gravitational Lensing, ESA, [Acessed 05/20/23], [https://esahubble.org/wordbank/gravitational-lensing/#:~:text=Gravitational%20lensing%20occurs%20when%20a,accordingly%20called%20a%20gravitational%20lens.]
Gravitational lensing, Hubblesite NASA, [Acessed 05/20/23], [https://hubblesite.org/contents/articles/gravitational-lensing]
Spyglasses into the Universe: gravitational lenses, ESA, [Acessed 05/20/23], [https://esahubble.org/science/gravitational_lensing/]
Different types of gravitational lenses, ESA, [Acessed 05/20/23], [https://esahubble.org/images/heic0404b/]
R. J. A., Lambourne., Relativity, Gravitation and Cosmology, Cambridge University Press, [2010], ISBN 978 0 521 13138 4
Gravitational lensing, University of California, Berkeley, [Acessed 05/20/23], [https://w.astro.berkeley.edu/~jcohn/lens.html]
Discoveries - Highlights | Shining a Light on Dark Matter, Hubble Space Telescope, NASA, [Acessed 05/20/23], [https://www.nasa.gov/content/discoveries-highlights-shining-a-light-on-dark-matter]
G. Gilmore., G. Tausch-Pebody., The 1919 eclipse results that verified general relativity and their later detractors: a story re-told, Royal Society Journal of the History of Science, [2021] https://royalsocietypublishing.org/doi/10.1098/rsnr.2020.0040]
Join our Space Forums to keep talking space on the latest missions, night sky and more! And if you have a news tip, correction or comment, let us know at: community@space.com.
Get the Space.com Newsletter
Breaking space news, the latest updates on rocket launches, skywatching events and more!
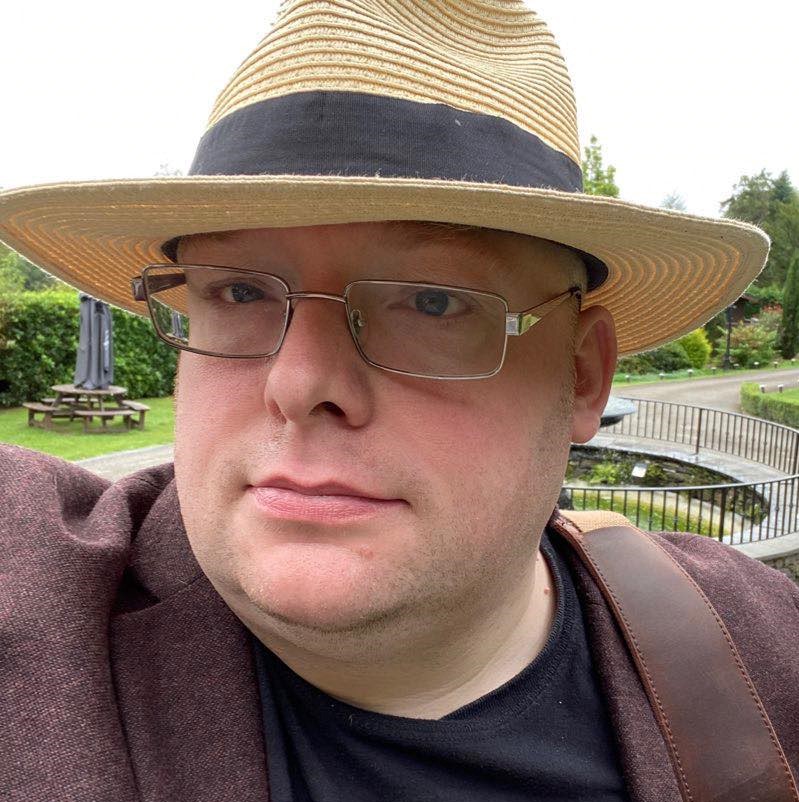
Robert Lea is a science journalist in the U.K. whose articles have been published in Physics World, New Scientist, Astronomy Magazine, All About Space, Newsweek and ZME Science. He also writes about science communication for Elsevier and the European Journal of Physics. Rob holds a bachelor of science degree in physics and astronomy from the U.K.’s Open University. Follow him on Twitter @sciencef1rst.