
What is an attosecond? A physical chemist explains the tiny time scale behind Nobel Prize-winning research
How long is an attosecond, and what can these infinitesimally short pulses tell researchers about the nature of matter?
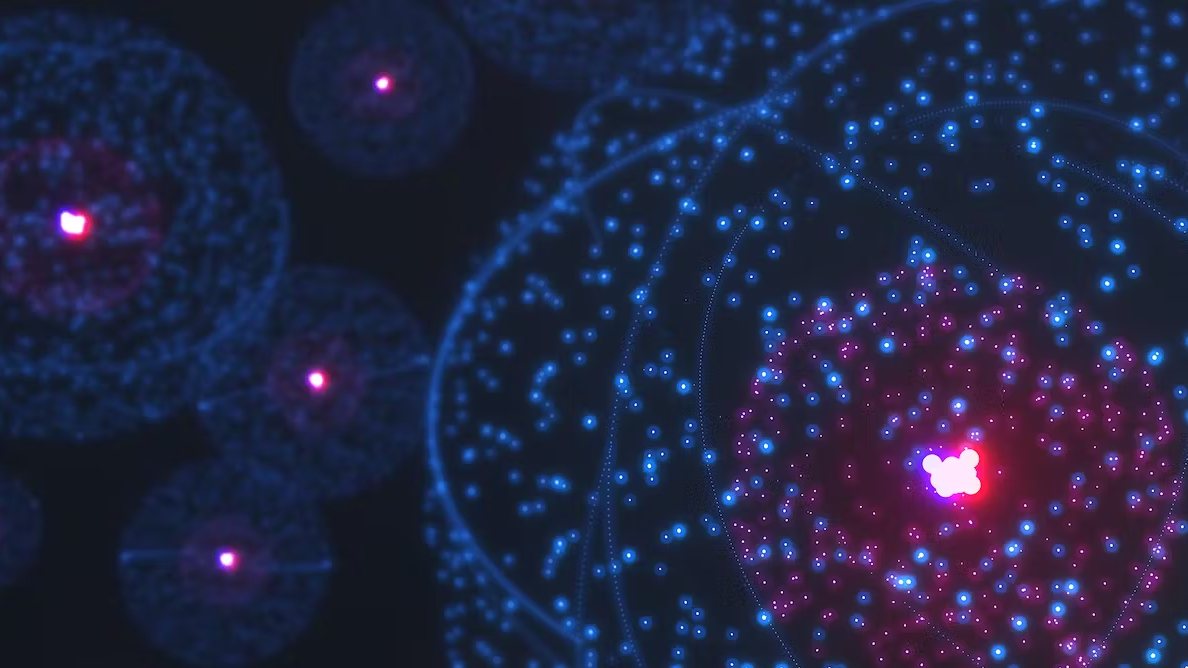
A group of three researchers earned the 2023 Nobel Prize in physics for work that has revolutionized how scientists study the electron – by illuminating molecules with attosecond-long flashes of light. But how long is an attosecond, and what can these infinitesimally short pulses tell researchers about the nature of matter?
I first learned of this area of research as a graduate student in physical chemistry. My doctoral adviser’s group had a project dedicated to studying chemical reactions with attosecond pulses. Before understanding why attosecond research resulted in the most prestigious award in the sciences, it helps to understand what an attosecond pulse of light is.
How long is an attosecond?
“Atto” is the scientific notation prefix that represents 10-18, which is a decimal point followed by 17 zeroes and a 1. So a flash of light lasting an attosecond, or 0.000000000000000001 of a second, is an extremely short pulse of light.
In fact, there are approximately as many attoseconds in one second as there are seconds in the age of the universe.
Previously, scientists could study the motion of heavier and slower-moving atomic nuclei with femtosecond (10-15) light pulses. One thousand attoseconds are in 1 femtosecond. But researchers couldn’t see movement on the electron scale until they could generate attosecond light pulses – electrons move too fast for scientists to parse exactly what they are up to at the femtosecond level.
Attosecond pulses
The rearrangement of electrons in atoms and molecules guides a lot of processes in physics, and it underlies practically every part of chemistry. Therefore, researchers have put a lot of effort into figuring out how electrons are moving and rearranging.
Get the Space.com Newsletter
Breaking space news, the latest updates on rocket launches, skywatching events and more!
However, electrons move around very rapidly in physical and chemical processes, making them difficult to study. To investigate these processes, scientists use spectroscopy, a method of examining how matter absorbs or emits light. In order to follow the electrons in real time, researchers need a pulse of light that is shorter than the time it takes for electrons to rearrange.
As an analogy, imagine a camera that could only take longer exposures, around 1 second long. Things in motion, like a person running toward the camera or a bird flying across the sky, would appear blurry in the photos taken, and it would be difficult to see exactly what was going on.
Then, imagine you use a camera with a 1 millisecond exposure. Now, motions that were previously smeared out would be nicely resolved into clear and precise snapshots. That’s how using the attosecond scale, rather than the femtosecond scale, can illuminate electron behavior.
Attosecond research
So what kind of research questions can attosecond pulses help answer?
For one, breaking a chemical bond is a fundamental process in nature where electrons that are shared between two atoms separate out into unbound atoms. The previously shared electrons undergo ultrafast changes during this process, and attosecond pulses made it possible for researchers to follow the real-time breaking of a chemical bond.
The ability to generate attosecond pulses – the research for which three researchers earned the 2023 Nobel Prize in physics – first became possible in the early 2000s, and the field has continued to grow rapidly since. By providing shorter snapshots of atoms and molecules, attosecond spectroscopy has helped researchers understand electron behavior in single molecules, such as how electron charge migrates and how chemical bonds between atoms break.
On a larger scale, attosecond technology has also been applied to studying how electrons behave in liquid water as well as electron transfer in solid-state semiconductors. As researchers continue to improve their ability to produce attosecond light pulses, they’ll gain a deeper understanding of the basic particles that make up matter.
Join our Space Forums to keep talking space on the latest missions, night sky and more! And if you have a news tip, correction or comment, let us know at: community@space.com.
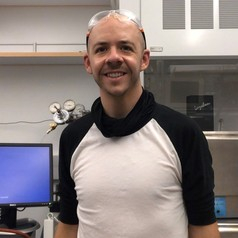
Aaron Harrison is an Assistant Professor of Chemistry at Austin College. His research in physical chemistry focuses on molecular photochemistry, spectroscopy, and computational chemistry.
-
OmShankar This article just rephrases more of the same information. How is an Attosecond pulse created? How is it used? None of that. Even after this article, we are just to take for granted that this is possible, without any explanation. Great.Reply -
Danny1961# Ok...So to create an attosecond pulse, The process is meticulous and requires precise control of laser pulses. First, an intense femtosecond laser pulse is generated, which typically falls within the visible or near-infrared spectrum.Reply
High Harmonic Generation (HHG): The process primarily used for creating attosecond pulses is High Harmonic Generation (HHG). In this method, the intense femtosecond laser pulse is focused onto a noble gas target, usually argon or helium.
Nonlinear Interaction: As the laser pulse interacts with the gas atoms, it causes a nonlinear response. The high intensity of the laser field leads to the generation of odd harmonics in the extreme ultraviolet (XUV) and soft X-ray regions.
Attosecond Pulse Formation: Due to the short duration of the driving laser pulse, the harmonic generation process produces bursts of XUV or soft X-ray radiation that are incredibly short, typically in the attosecond range (1 attosecond = 10^-18 seconds).
Isolation and Characterization: Sophisticated techniques are then employed to isolate and characterize these attosecond pulses. Techniques like attosecond streaking or attosecond transient absorption spectroscopy are used to study their properties.They have several applications sir,
Studying Electron Dynamics: Attosecond pulses allow physicists to probe and control electron motion in atoms and molecules. This is crucial for understanding fundamental processes in chemistry and physics.
Attosecond Pump-Probe Experiments: Researchers use attosecond pulses to perform pump-probe experiments, where one attosecond pulse (the pump) excites a sample, and another attosecond pulse (the probe) measures its response. This enables the study of ultrafast processes, such as electron migration and charge transfer.
Imaging Electronic Structure: Attosecond pulses can be used in imaging techniques like attosecond photoelectron spectroscopy to map the electronic structure of atoms and molecules.
Real-Time Observations: Attosecond pulses enable real-time observation of electronic motion, making it possible to investigate phenomena like electron tunneling and ionization with unprecedented temporal resolution.