Dark matter atoms may form shadowy galaxies with rapid star formation
In new simulations, 'dark atoms' formed dark stars and could even trigger the formation of black holes.
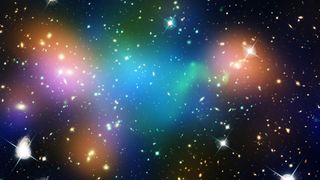
Dark matter, the invisible material that makes up the vast majority of the universe's mass, may collect itself to form atoms, a new simulation shows.
Those "dark atoms" might radically alter the evolution of galaxies and the formation of stars, giving astronomers a new opportunity to understand this mysterious substance.
Dark matter makes up over 80% of the mass of every galaxy and cluster of galaxies in the universe. All of our observations suggest that dark matter is some new kind of particle, one that does not interact with normal matter or even with light. We can identify dark matter only through its gravitational interactions with everything else. Whatever dark matter is, it is beyond our current understanding of physics. But it still has mass, so it still has gravity.
We do not yet know if dark matter is simple or complex. It may be made of only one kind of particle that dominates the universe and barely interacts with even itself. Or it may be made of multiple types of particles, with as rich a variety as we see in normal matter. Beyond that, we know of only four fundamental forces of nature: gravity, electromagnetism, the strong nuclear force and the weak nuclear force. But there may be additional forces that operate only among the dark matter particles and do not interfere with normal matter at all.
Related: What is dark matter?
Getting together
The concept of additional dark matter particles and dark forces isn't as far-fetched as it may seem. Our understanding of physics is built on symmetries, which are deep mathematical relationships between particles. It could very well be that there are additional symmetries in the laws of nature that make dark matter a twin of normal matter and that, for every kind of interaction that normal matter can participate in, there is a counterpart in the dark sector.
For example, with normal matter, we can build simple atoms: a proton and an electron bound together, with the photon, the carrier of the electromagnetic force, mediating the interaction. We could also have a dark matter version of that same structure, with a dark proton bound to dark electrons via dark photons: dark atoms.
Get the Space.com Newsletter
Breaking space news, the latest updates on rocket launches, skywatching events and more!
Atomic dark matter would behave much differently than dark matter composed of only a single particle. Most importantly, simple dark matter would have a very difficult time clumping up, only doing so slowly over hundreds of millions of years. Normal matter collects in those smooth pools of dark matter to form galaxies, but otherwise, the two lead separate lives. Atomic dark matter, however, could form its own shadowy galaxies — disk-like structures that mimic the size and layout of visible galaxies.
A team of astrophysicists used this intriguing possibility to simulate the evolution of galaxies and see what observable differences might arise. They allowed the atomic dark matter to evolve according to its own forces and then examined how those new structures would affect visible galaxies through the new arrangement of gravity. They published their results in the online preprint database arXiv in April.
Related: If dark matter is 'invisible,' how do we know it exists?
Stellar sabotage
The researchers found that even a small amount of atomic dark matter — as little as 6% of all the dark matter in the universe, leaving the rest to be simple — was enough to radically alter the evolution of galaxies. Because the atomic dark matter could interact, it could easily clump together by losing energy through the emission of some form of dark radiation. The simulations revealed that a "dark disk" quickly appeared within each galaxy, with the spin of the disk closely matching that of the visible, normal components.
From there, the atomic dark matter continued to clump, just like normal gas clumps into clouds and, eventually, stars. In the simulation, the atomic dark matter formed dark stars of its own and could even trigger the formation of its own black holes. Those clumps then sank into the core of the galaxy, where the density increased.
With all that extra gravity, star formation in the cores of galaxies kicked into overdrive, producing stars at a much faster rate than in galaxies with simple dark matter. These simulations actually ruled out some models of atomic dark matter, because those models caused their galaxies to run out of new material for making stars far too quickly.
But some models survived current observational limits, allowing for the continued possibility of atomic dark matter. The researchers hope that further theoretical and experimental studies will shed light on the plausibility of this intriguing form of exotic matter. For example, because atomic dark matter clumps so efficiently, we might be able to spot dense, star-like clumps with upcoming gravitational microlensing studies with NASA's Nancy Grace Roman Space Telescope.
Join our Space Forums to keep talking space on the latest missions, night sky and more! And if you have a news tip, correction or comment, let us know at: community@space.com.
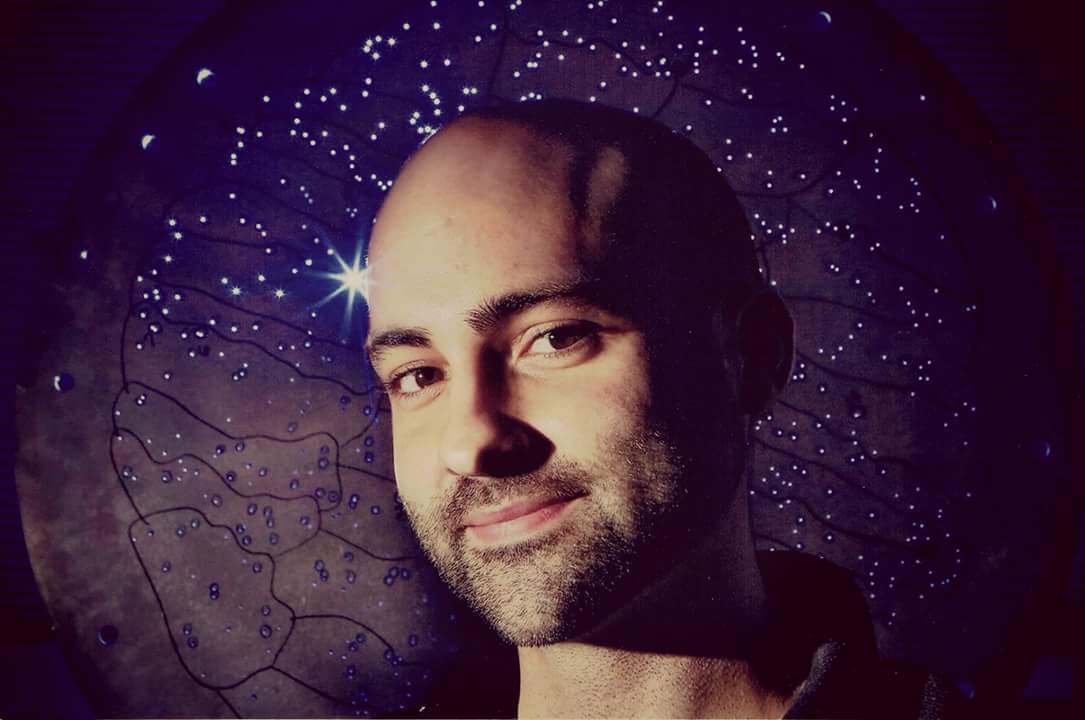
Paul M. Sutter is an astrophysicist at SUNY Stony Brook and the Flatiron Institute in New York City. Paul received his PhD in Physics from the University of Illinois at Urbana-Champaign in 2011, and spent three years at the Paris Institute of Astrophysics, followed by a research fellowship in Trieste, Italy, His research focuses on many diverse topics, from the emptiest regions of the universe to the earliest moments of the Big Bang to the hunt for the first stars. As an "Agent to the Stars," Paul has passionately engaged the public in science outreach for several years. He is the host of the popular "Ask a Spaceman!" podcast, author of "Your Place in the Universe" and "How to Die in Space" and he frequently appears on TV — including on The Weather Channel, for which he serves as Official Space Specialist.
-
Unclear Engineer Glad to see this type of thinking being considered and tested. Considering the diversity of the 20% of matter that we can see, it seems very naive to expect that the 80% that we cannot see, but infer (from gravitational effects), would be only one simple thing (particle type or whatever).Reply
On the other hand, if we can simply assume that there is the same complexity, or maybe 4 times the complexity that we see in visible matter, then we can use all of that as "fitting parameters" for making the Big Bang Theory match whatever observations we make in the future that otherwise would seem to undercut its credibility.
It is not just important, it is necessary to constantly keep in mind what is an observation and what is an assumption when doing this sort of thinking. Otherwise, people begin to think that they have "proven" their thinking is correct simply because they have managed to not violate the observations with what might be a wholly unrealistic set of assumptions that are only internally consistent, not really proven. (That is where I am concerned that the BBT has already gone - requiring that 95% of its mass and energy are based on assumptions, rather than detected materials or forces that obey known physical mechanisms.)
In the case of the BBT, I don't think that there is even a completely consistent set of assumptions. So, thinking that is broad enough to question some of those assumptions is something that I welcome, especially if it looks at some of the potentials for inconsistencies. -
rod Well, this is an interesting report on DM. The reference paper cited is 23-page PDF. I note from the paper:Reply
Draft version April 21, 2023
Typeset using LATEX twocolumn style in AASTeX631
Simulating Atomic Dark Matter in Milky Way Analogues, https://arxiv.org/abs/2304.09878
"ABSTRACT Dark sector theories naturally lead to multi-component scenarios for dark matter where a subcomponent can dissipate energy through self-interactions, allowing it to eciently cool inside galaxies.
We present the rst cosmological hydrodynamical simulations of Milky Way analogues where the
majority of dark matter is collisionless Cold Dark Matter (CDM), but a sub-component (6%) is strongly
dissipative minimal Atomic Dark Matter (ADM)."
"1. INTRODUCTION...Dark sectors frequently include a fraction of DM with dissipative self-interactions, which can cool and clump in opposition to the purely gravitational dynamics of CDM. A useful benchmark model for such scenarios is Atomic Dark Matter (ADM), which consists of a dark proton, p0, and dark electron, e0, that interact through a massless dark photon with coupling, 0 (Kaplan et al. 2009). The ADM can form a dark hydrogen bound-state and radiatively cools in direct analogy to the Standard Model. We assume a minimal model that has no dark nuclear physics and only couples to the Standard Model through gravity. Moreover, we assume that the ADM abundance is set asymmetrically, so that the abundance of dark anti-particles is negligible (Zurek 2013; Kaplan et al. 2011)."
I found 7 references to assume or assumed in the paper. Also some other good stuff :)
"On cosmic scales, ADM manifests through dark acoustic oscillations and contributions to Ne (Cyr-Racine
et al. 2014; Gurian et al. 2022a). Current cosmological constraints allow for ADM to comprise & O(10%) of
the DM for a wide range of parameters (Bansal et al. 2022b,a). On much smaller scales, the signatures of
an ADM sub-component can potentially be spectacular. ADM gas clouds can collapse and condense into dark compact objects, giving rise to dark white dwarfs (Ryan & Radice 2022) and non-stellar-mass black holes (Shandera et al. 2018; Fernandez et al. 2022), as well as mirror (neutron) stars (Curtin & Setford 2020a,b; Hippert et al. 2022b,a) (if there is dark nuclear physics)."
It is time to show a database listing all DM stuff now :) -
Pogo Dark atoms? Is that with dark protons, dark neutrons, dark electrons, dark quarks? Emitting dark photons and dark neutrinos? And dark antimatter? Interesting indeed!Reply -
bwana4swahili More wasted research $$$'s and resources on dark matter which in all probability doesn't exist! Whatever happened to the KISS principle in scientific research!?Reply -
Atlan0001 I have a tendency to forget the dark part, and this article makes me want to forget it even more since we detect it via its gravitational effect, which is a universal effect, a uniform effect . . . regardless of degree.Reply
Light follows spacetime curvature. There may be more paths to the universe than just a light following 1-dimensional curvature and gravity may deal in one or more of those other paths (one or more other dimensions of pathing). Thus, the only reason for the darkness may be that we just don't observe that fold, or level, or whatever, in spacetime from our position, or velocity, or horizon. If we were travelers in and through the universe, we might observe and reach far more of the universe -- finding a far different, possibly far larger, far wider and deeper, universe (thus possibly many more universes belonging to our own local all-in-one multiverse universe) -- than we can possibly observe from Earth . . . or any one place in it (again, once more, "the map (the light map arrived to Earth) is not the territory").