Cracking the Code of Pre-Earthquake Signals
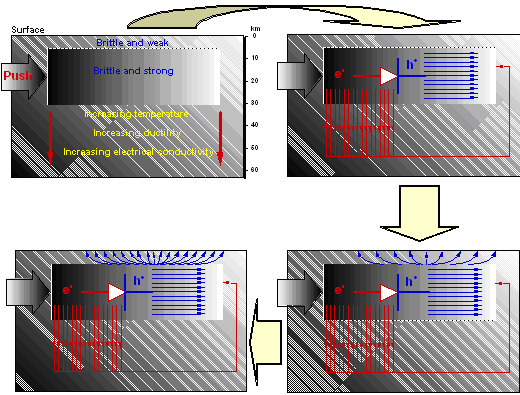
Our Earth is a restless planet. Occasionally - quite often, in some regions of the world - the restlessness turns deadly. Of all natural hazards, earthquakes are the most feared. They are feared because they seem to strike so unpredictably. Yet, for centuries, and even millennia, people living in seismically active regions have noted premonitory signals. The historical records talk of changes of the water level in wells, of strange weather, of ground-hugging fog, of unusual behavior of animals (both domestic and wild) that seem to feel the approach of a major earthquake. With the advent of modern science and technology the list of premonitory signals has become even longer. Among them are
- Sporadic emissions of low to ultralow-frequency electromagnetic radiation from the ground
- Occasional local magnetic field anomalies reaching a strength of half a percent of the Earth's main dipole field
- Changes in the lower atmosphere that are accompanied by the formation of haze and a reduction of moisture in the air
- Large patches, often tens to hundreds of thousands of square kilometers in size, seen in night-time infrared satellite images where the land surface temperature seems to fluctuate rapidly
- Passing perturbations in the ionosphere at 90 - 120 km altitude that affect the transmission of radio waves
Electrical rocks
We normally think of rocks as being good insulators, i.e., rocks are very poor at conducting electrical currents. However, in rocks whose minerals contain peroxy bonds, a time bomb is ticking. When these rocks are subjected to stress, the peroxy bonds break and suddenly mobile electronic charge carriers appear, so-called defect electrons that live and travel in the valence band of the constituent minerals. These charge carriers are also called positive holes or p-holes for short.
Looking back over the 30 years since their discovery, I am surprised to note that I always returned to these strange and elusive charge carriers. I tried to understand their nature and to predict their behavior. The breakthrough came when I realized that these p-holes could be activated by stress. This put me squarely on the track to study earthquake-related phenomena.
Still, it took several years and several wrong starts until I was able to conceive an experiment that is amazingly simple and, at the same time, full of surprises. In figure 1, a 1.2-meter long slab of granite which, together with my coworkers Dr. Akihiro Takeuchi and Dr. Bobby Lau, I had fitted with copper electrodes at both ends to measure currents and with a capacitor plate on the top surface to measure potentials. We inserted one end of the slab into a powerful press, but insulated it from the pistons. Then, we started to squeeze. We squeezed the rock many times and recorded the currents that started to flow out of both ends.
The experiment showed that the stressed volume of rock becomes a source of electronic charge carriers, p-holes and electrons. Since p-holes and electrons flow out in opposite directions, something important must happen at the boundary between the stressed and unstressed rock. The boundary allows p-holes to pass but blocks electrons. It therefore acts like a diode in a transistor. Obviously the unstressed granite is capable of conducting p-holes, meaning that it behaves like a p-type semiconductor. The electrons can flow out of the stressed rock volume only if there is an n-type connection - in our case the copper electrode.
Next we may wonder how long such currents can flow if we keep the load constant. We did a similar stress test with gabbro, another igneous rock. Upon keeping the load constant for 30 minutes, the two currents flow with barely any loss in their intensities. Even keeping the load constant for 12 hours leads to not more than a 15-20% reduction of the currents. This shows that, once activated, the p-holes and electrons in the stressed rock volume have a very long lifetime.
Breaking space news, the latest updates on rocket launches, skywatching events and more!
Use for earthquake prediction
How can we apply this new knowledge to earthquakes and to those hidden processes that take place deep in the Earth's crust before tectonic stresses reach a critical level where rupture occurs and the ground starts to shake?
Though we stand only at the beginning of a long road to discoveries yet to come, we can already project some of our findings into geophysical reality.
In figure 2, you can see a sketch of a very simplified model depicting a section through the Earth's crust where tectonic forces begin to act on a large block of strong, rigid rocks, maybe 100 - 1000 km wide, 20 km thick and 50 - 10 km in the thrust direction. As stresses build up from the left, they cause plastic deformation propagating toward the right. The volume of rocks undergoing deformation becomes the source of p-holes and electrons. The p-holes can flow out horizontally. The electrons can flow out only if they can connect downward into the deeper, hotter and, hence, n-type conducting portions of the lower crust.
In this model we obviously neglect to take into account the role of water which fills faults that deeply dissect the Earth's crust in all tectonically active regions. Faults filled with water or brines will introduce complications, but we already know from laboratory experiments that water may short-circuit the p-hole conduction through the rocks but it does not "kill" it. Therefore we can cautiously go ahead and project some of the consequences of the p-hole activation in rocks that experience ever-increasing levels of stress.
One of these consequences is that the p-hole current flowing horizontally through the crust should couple to the electron current flowing downward. The coupling is provided by their respective electric fields. As a result both currents can be expected to fluctuate just like the p-hole and electron currents did in our laboratory experiments. Fluctuating currents are a source of low frequency electromagnetic (EM) radiation. Thus our model, simple as it may be, points to the possibility that the often reported pre-earthquake low frequency EM emissions arise from ground currents flowing deep in the Earth's crust. The ground currents may be very powerful. For instance, taking the currents flowing out of the squeezed end of the granite slab in our experiment, we may ask what would be the current flowing out of a cubic kilometer of granite or gabbro in the crust, all other conditions being the same. The answer is a surprisingly large value, somewhere between 100,000 and 1,000,000 amperes. Since huge volumes of rocks - tens of thousands of cubic kilometers - come under increasing stress during the build-up of large earthquakes, the ground currents could indeed be enormous. Looking at it from a different perspective, we can say that, even if most of the currents generated in the ground are short-circuited or annihilated by other factors, those that remain might still reach impressively large values.
Atmospheric disturbances
Another result of stress-activated currents flowing in the ground would be that some p-holes will reach the surface of the Earth. They would change the ground potential over large areas, making it more positive relative to the surrounding areas. This would have many consequences, of which I only want to mention one.
Roughly 90 - 120 km above the Earth's surface the ionosphere begins, which is composed of a highly dynamic plasma of electrons and ions generated under the daily assault of extreme ultraviolet radiation from the Sun, solar wind bombardment, and cosmic rays. If the land surface below becomes increasingly positive, this plasma sheet will react. Maybe the source for the well-documented pre-earthquake ionospheric perturbations lies in the activation of p-holes deep in the Earth's crust and the mischief that they play at the Earth's surface.
In hindsight it is quite amazing to see how a line of basic research that, at its outset three decades ago seemed to have no connection whatsoever to the origin of life and to earthquakes, has become a treasure drove of insights and discoveries. It is certainly too early to say that earthquake prediction is just around the corner. However, I feel confident that the discovery of p-holes in rocks and their activation by stress represents a crucial step toward cracking the code of the Earth's multifaceted pre-earthquake signals.
Join our Space Forums to keep talking space on the latest missions, night sky and more! And if you have a news tip, correction or comment, let us know at: community@space.com.