James Webb Space Telescope could target tiny bright galaxies to shine light on dark matter
"The discovery of patches of small, bright galaxies in the early universe would confirm that we are on the right track with the cold dark matter model."
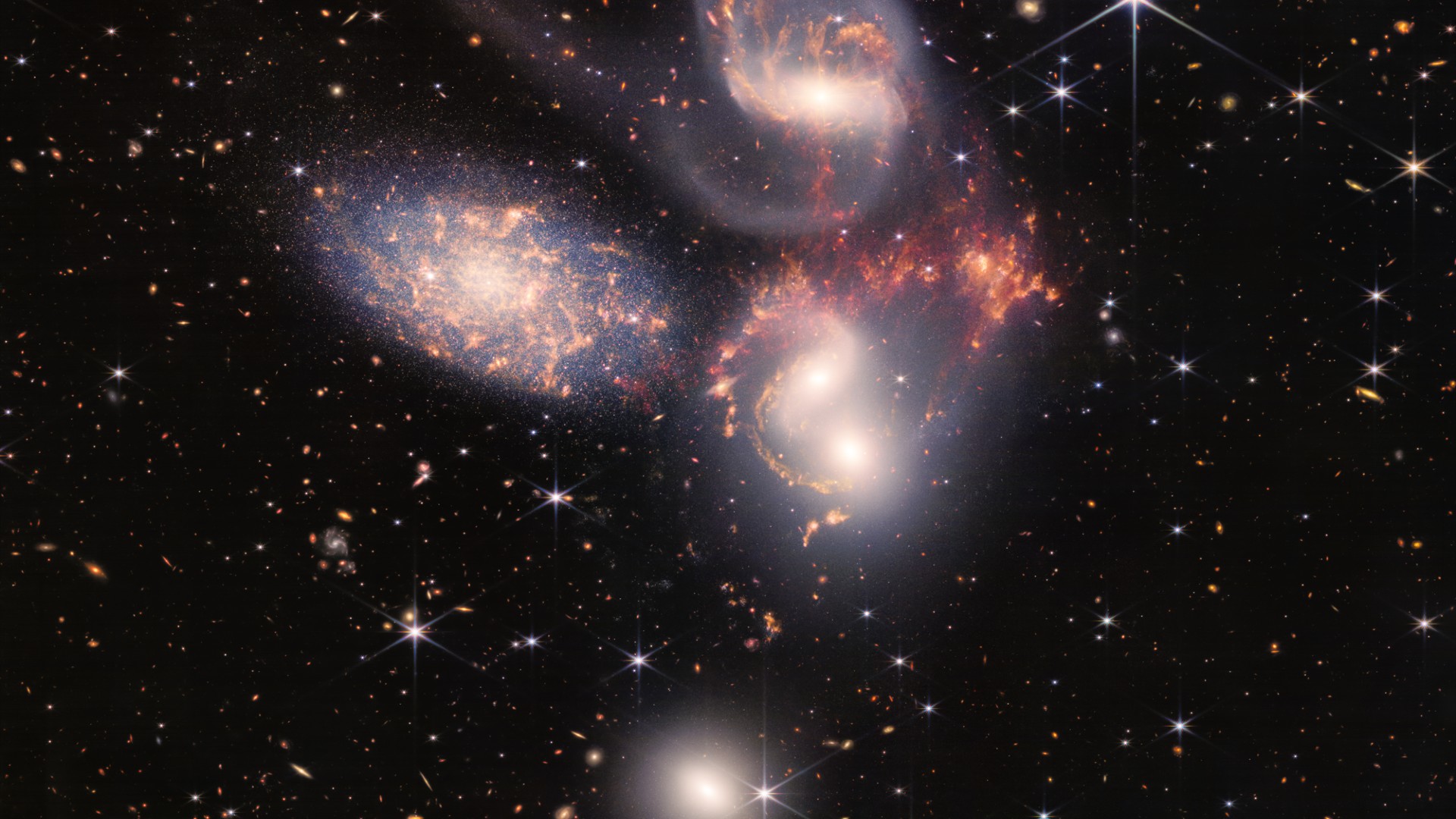
If the James Webb Space Telescope (JWST) investigates tiny and bright galaxies in the early universe, it may be able to to shine a light on dark matter — the universe's most mysterious stuff.
Such is the conclusion reached by scientists from the University of California, who ran a simulation of the cosmos that tracks the formation of small galaxies — starting as far back as shortly after the Big Bang. This appears to have upped the ante for the JWST.
Small galaxies, also known as dwarf galaxies, are distributed throughout the cosmos, and scientists have suggested they may represent some of the earliest galaxies to have formed. This means dwarf galaxies have often been considered key in studying the origins and evolution of the universe.
The problem has been, however, that these galaxies don't always match what astronomers expect to observe. For instance, some spin faster than expected, and others are less dense than simulations suggest they should be. This is where dark matter comes in.
These puzzling contradictions, scientists think, could exist because researchers haven't factored into their simulations the combination of gas and dark matter.
Related: James Webb Space Telescope observes 19 intricate galaxy structures in stunning detail (images)
The team's new simulation thus factored in those interactions between dark matter and gas, finding that early galaxies that are created smaller and much brighter than those in simulations that neglect the interplay. The scientists also saw the galaxies growing more rapidly than other teams have seen.
Get the Space.com Newsletter
Breaking space news, the latest updates on rocket launches, skywatching events and more!
Hence, the UCLA team thinks astronomers should start hunting small, early galaxies that are much brighter than expected using the JWST and other telescopes. Should these galaxies fail to turn up, well, then something could actually just be wrong with our theories of dark matter.
In the dark about dark matter
Dark matter is such a headache for scientists because it doesn't interact with light, which makes it effectively invisible to us.
The matter that makes up stars, gas, planets, our bodies, your next door cat and pretty much everything you see around you is comprised of atoms made of electrons, protons and neutrons. These are called "baryons," and they do interact with light. Thus, scientists realized, dark matter must be made up of something else — something "non-baryonic."
All of this means that, despite the fact that dark matter accounts for around 85% of the mass in the universe, scientists can't detect it directly and have no solid idea what it is made of.
Because dark matter has mass, it does interact with gravity. That means its presence can be inferred by how these gravitational effects impact baryonic matter and indeed light.
The whole concept of dark matter was initially postulated, in fact, because galaxies are spinning so rapidly that the gravitational influence of their baryonic matter alone couldn't prevent them from flying apart. It is the influence of unseen dark matter that gravitationally "glues" galaxies together, scientists believe.
Scientists further posit that most galaxies are surrounded by vast haloes of dark matter that extend way beyond their visible star, gas and dust content. They also think these haloes may have been integral to the galaxies' formation and evolution.
In the currently favored model of universal evolution, the "standard cosmological model," the gravitational influence of dark matter clumps that existed in the universe 13 billion years ago managed to draw in baryonic matter made of
normal old atoms.
Once this "ordinary matter" grew massive enough, it collapsed to birth the first stars. Along with dark matter, these first stars drew in more baryonic matter, creating the galaxies around them.
The standard model features a form of dark matter called "cold dark matter," which gets its name not because it is chilly but because it moves slower than the speed of light (heat being a measure of how fast particles are moving). The gathering of stars and galaxies in the standard cosmological model would also be slow if they were dependent on cold dark matter.
Baryonic matter in the form of hydrogen and helium gas from the Big Bang would have streamed past those slow-moving dark matter clumps at supersonic speeds in this early stage of the universe's history. That is, until the matter got ultimately ensnared, then collected together to form galaxies.
"Indeed, in models that do not take streaming into account, this is exactly what happens," Claire Williams, team member and a doctoral student at UCLA, said in a statement. "Gas is attracted to the gravitational pull of dark matter, forms clumps and knots so dense that hydrogen fusion can occur, and thus forms stars like our sun."
Williams and colleagues found that, when this so-called streaming effect between dark and ordinary matter is accounted for in their simulation, part of the aptly named "Supersonic Project," gas landed far from dark matter and growing galaxies. This prevented the immediate formation of stars.
Millions of years after this, the gas eventually fell back into the galaxies, triggering an intense spate of star formation called "starburst," creating galaxies that had many more young, hot stars than ordinary small galaxies. For a time, those starburst galaxies should've shone much brighter than other galaxies.
"While the streaming suppressed star formation in the smallest galaxies, it also boosted star formation in dwarf galaxies, causing them to outshine the non-streaming patches of the universe," Williams explained. "We predict that the JWST telescope will be able to find regions of the universe where galaxies will be brighter, heightened by this velocity.
"The fact that they should be so bright might make it easier for the telescope to discover these small galaxies, which are typically extremely hard to detect only 375 million years after the Big Bang."
The fact that dark matter is effectively invisible means these small, bright galaxies in the early universe would make a good proxy by which to test the cold dark matter concept. Failure to detect them may mean scientists have to turn to other theories.
"The discovery of patches of small, bright galaxies in the early universe would confirm that we are on the right track with the cold dark matter model because only the velocity between two kinds of matter can produce the type of galaxy we’re looking for," Smadar Naoz, Supersonic team leader and a UCLA physics and astronomy professor, said in the statement. "If dark matter does not behave like standard cold dark matter and the streaming effect isn’t present, then these bright dwarf galaxies won’t be found, and we need to go back to the drawing board."
The team's research is published in The Astrophysical Journal Letters,
Join our Space Forums to keep talking space on the latest missions, night sky and more! And if you have a news tip, correction or comment, let us know at: community@space.com.
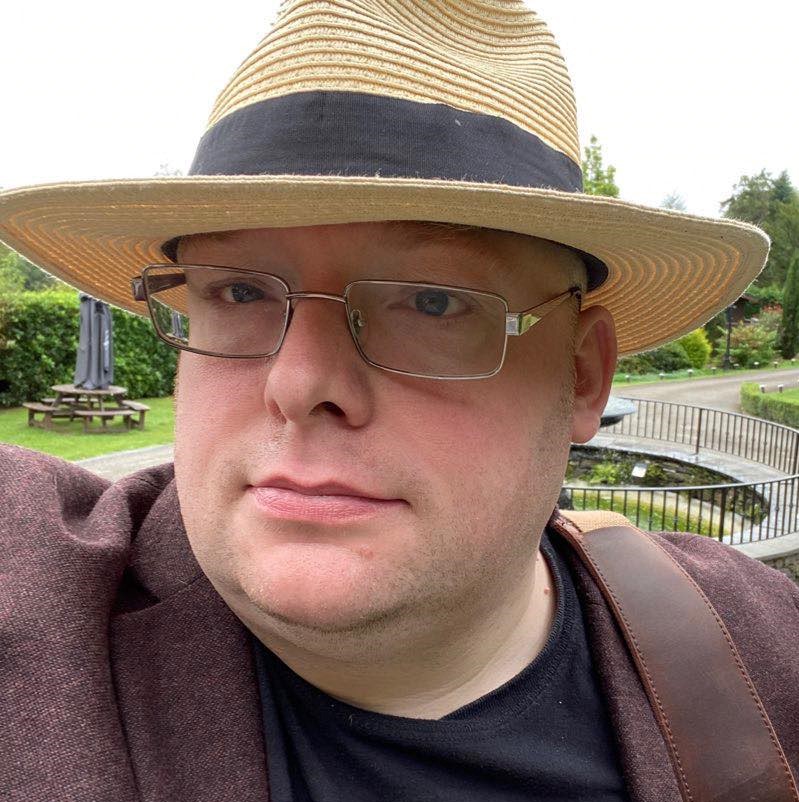
Robert Lea is a science journalist in the U.K. whose articles have been published in Physics World, New Scientist, Astronomy Magazine, All About Space, Newsweek and ZME Science. He also writes about science communication for Elsevier and the European Journal of Physics. Rob holds a bachelor of science degree in physics and astronomy from the U.K.’s Open University. Follow him on Twitter @sciencef1rst.
-
rod Space.com reported, "Small galaxies, also known as dwarf galaxies, are distributed throughout the cosmos, and scientists have suggested they may represent some of the earliest galaxies to have formed. This means dwarf galaxies have often been considered key in studying the origins and evolution of the universe. The problem has been, however, that these galaxies don't always match what astronomers expect to observe. For instance, some spin faster than expected, and others are less dense than simulations suggest they should be. This is where dark matter comes in. These puzzling contradictions, scientists think, could exist because researchers haven't factored into their simulations the combination of gas and dark matter."Reply
My note. Okay, apparently DM in the early universe, simulations and modeling must be very flexible to show DM :) Concerning redshifts in this report, z about 10 to 12 in the paper reference. Cosmology calculators show the age of the universe and distances too. https://lambda.gsfc.nasa.gov/toolbox/calculators.html
"The age at redshift z was 0.478 Gyr. The light travel time was 13.244 Gyr." for z = 10 and for z=12, "The age at redshift z was 0.372 Gyr. The light travel time was 13.350 Gyr."
Comoving radial distances beyond 32 billion light-years from Earth today where space expands much faster than c velocity. The reference paper makes it clear that Population III stars remain undetected at large redshifts still.
"1. Introduction The James Webb Space Telescope (JWST) has opened a new window on the first galaxies in the Universe, allowing for new insights regarding their properties and formation. These galaxies at "cosmic dawn" host the yet-undetected first generation of stars (Population III), reionize the Universe, and enrich their surroundings with the first metals.", ref - https://iopscience.iop.org/article/10.3847/2041-8213/ad1491 -
Questioner Visible matter orbits the central black hole of its galaxy.Reply
How & why by what invisible physics does the DM do likewise?
DM is itself immune to gravity by all observations, meaning it has no apparent inertia.
When a galaxy orbits another galaxy how is it that the coincidental centering of both visible matter & DM remains generally intact, even though the DM has tremendously more mass (gravitational effect)?
Why does inertialess DM slave itself to visible matter's inertia?