Did a holographic phase transition in the early universe release gravitational waves?
If so, we should be able to detect these ripples in space-time in the not-too-distant future.
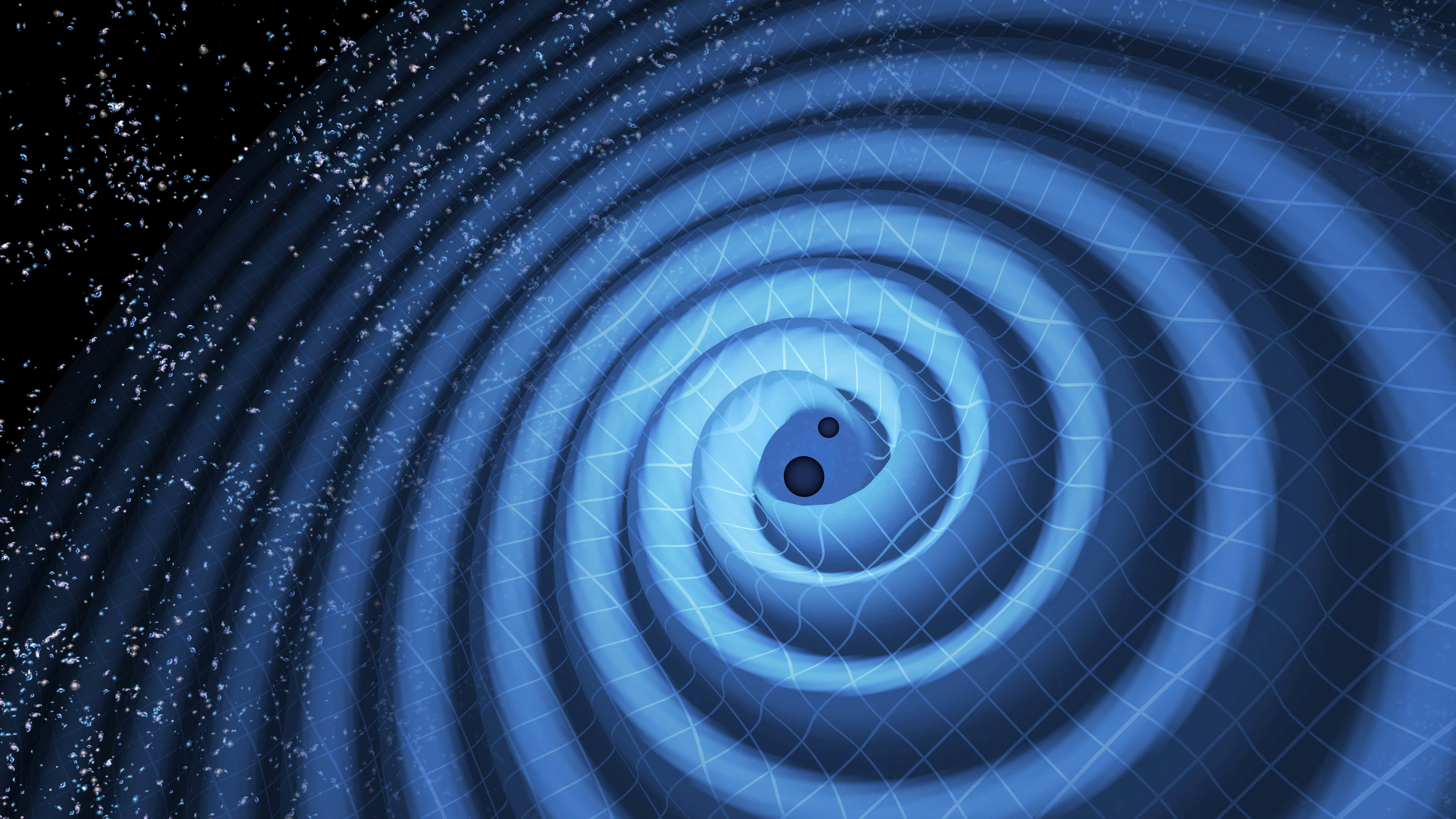
Paul M. Sutter is an astrophysicist at SUNY Stony Brook and the Flatiron Institute, host of Ask a Spaceman and Space Radio, and author of How to Die in Space. He contributed this article to Space.com's Expert Voices: Opinions and Insights.
As I'm sure you can understand, we don't know much about the extremely early universe.
We do suspect that it went through several major transformative epochs — called phase transitions — that eventually led to the universe that we know and love. Recently, a team of physicists have used one of the most powerful tools from string theory to tackle these phase changes, and revealed that we are on the cusp of directly detecting those events through their gravitational wave signature.
Related: Hunting gravitational waves: The LIGO laser interferometer project in photos
A new phase
There are four known forces of nature. Two of them are very familiar to you: the force of gravity and the electromagnetic force, which combined make up the bulk of our everyday experiences. A third, the strong nuclear force, is responsible for holding atomic nuclei together, but other than that, its extremely short range prevents it from doing much else of note. The fourth, the weak nuclear force, is what makes nuclear decay and reactions possible, which is pretty handy. But otherwise, that force just sits around minding its own business.
These four forces couldn't be any more different from each other, but one of the most remarkable insights of modern physics is that they might all be manifestations of the same force. I can't just call that unified force "the force," because the "Star Wars" universe already took that moniker, so we'll have to settle for "unified force."
We don't know if the unified force actually exists (or ever existed), but we have been able to merge two of the forces of nature together. Inside our high-energy particle collider experiments, the electromagnetic and weak nuclear forces merge together to become a unified "electroweak" force.
Get the Space.com Newsletter
Breaking space news, the latest updates on rocket launches, skywatching events and more!
And if our particle colliders can achieve this feat, then surely the universe can. When our cosmos was less than a second old, it was incredibly small, hot and dense. Small, hot and dense enough for the electroweak force to run wild. It was only once the universe expanded and cooled past this point that the forces could separate into their distinct identities.
The universe: Big Bang to now in 10 easy steps
Rough ride
In our simplest models, this transition was relatively smooth — an easy-peasy crossover from the electroweak regime to the universe of the separate electromagnetic and weak nuclear forces. But our simplest models of physics, while radically successful, do have their shortcomings. We're not able to explain all of the variety of physics in the universe (like, say, the mass of the neutrino and the presence of dark matter) with our simple models.
More complex (and more hypothetical) models of physics predict that the phase transition that ended the electroweak epoch was very violent. Instead of one steady universal transition, it was rough and bumpy. Bubbles of new forces formed, grew and merged in a violent release of energy, with the universe erupting in chaotic fury as the electroweak force separated from itself.
But beyond the ability to say "yup, it was crazy," the tools of modern physics aren't up to the task of describing that violent phase transition in any more detail. Our math just isn't good enough to track all the complex, strong forces as they transformed the universe.
String theory to the rescue.
Into the hologram
Well, not string theory per se. String theory is our attempt to describe a unified force (and explain all of physics with one single theory), but it hasn't been cracked — nobody has been able to solve the math of string theory in order to actually make predictions (which is kind of important for science).
But in the decades that physicists have been working to unravel the mysteries of string theory (pun intended), they stumbled across an apparently powerful technique. Some problems that seem intractably hard can be transformed into relatively simple easy-to-solve questions, then transformed back to get an answer.
The catch: you have to think in higher dimensions. In our case of trying to understand the nastiness of the early universe, the hard (and possibly impossible) math behind the physics of the phase transition can be transformed into a simpler problem involving general relativity — in five dimensions.
It doesn't make any sense. Why does this trick work? Why do electroweak problems in four dimensions become gravity problems in five? We don't know. But we do know that this approach just might be crazy enough to work, and a team of theoretical physicists have used this approach to model the physics of the early universe, as reported in a paper recently appearing on the preprint journal arXiv.
Armed with this newfangled "holographic" (as the technique is called, because it involves translating from one set of dimensions to another without losing information) trick, the theorists were able to track the formation of bubbles during the electroweak phase transition. They found that bubble formation and collision results in a tremendous release of gravitational waves.
Those ripples in space-time can persist to the present day. But even though they would've torn you up like a piece of paper when they first formed, nowadays they can barely nudge an atom. We don't yet have the sensitivity to detect them, but the researchers found that proposed space-based gravitational wave detectors, like the European Space Agency's Laser Interferometer Space Antenna mission, or LISA, will.
When LISA is fully operational (which will take another couple decades at least), it's possible that it could detect these faint gravitational waves that are left over from when the last of the four forces of nature split from each other. Without holographic theory, we would never have been able to make this sort of prediction, and the earliest and most violent epochs in the history of the cosmos would still remain a mystery.
Read more: "Gravitational waves from a holographic phase transition"
Follow us on Twitter @Spacedotcom or Facebook.
Join our Space Forums to keep talking space on the latest missions, night sky and more! And if you have a news tip, correction or comment, let us know at: community@space.com.
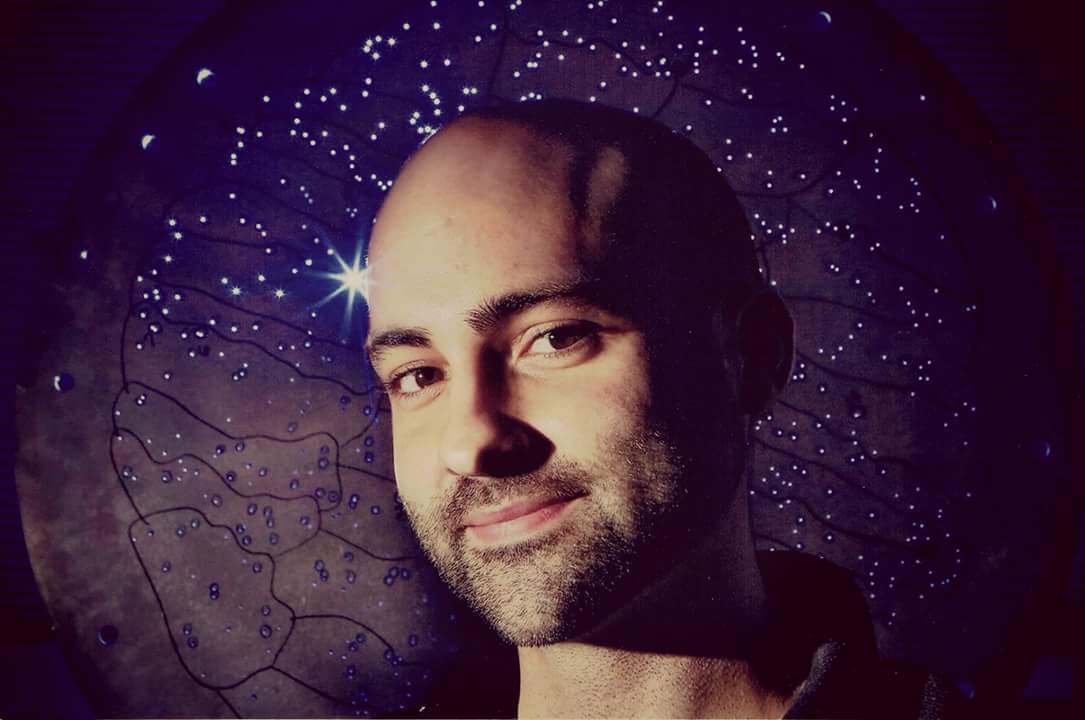
Paul M. Sutter is an astrophysicist at SUNY Stony Brook and the Flatiron Institute in New York City. Paul received his PhD in Physics from the University of Illinois at Urbana-Champaign in 2011, and spent three years at the Paris Institute of Astrophysics, followed by a research fellowship in Trieste, Italy, His research focuses on many diverse topics, from the emptiest regions of the universe to the earliest moments of the Big Bang to the hunt for the first stars. As an "Agent to the Stars," Paul has passionately engaged the public in science outreach for several years. He is the host of the popular "Ask a Spaceman!" podcast, author of "Your Place in the Universe" and "How to Die in Space" and he frequently appears on TV — including on The Weather Channel, for which he serves as Official Space Specialist.