
Dusty Plasma in the Universe and in the Laboratory
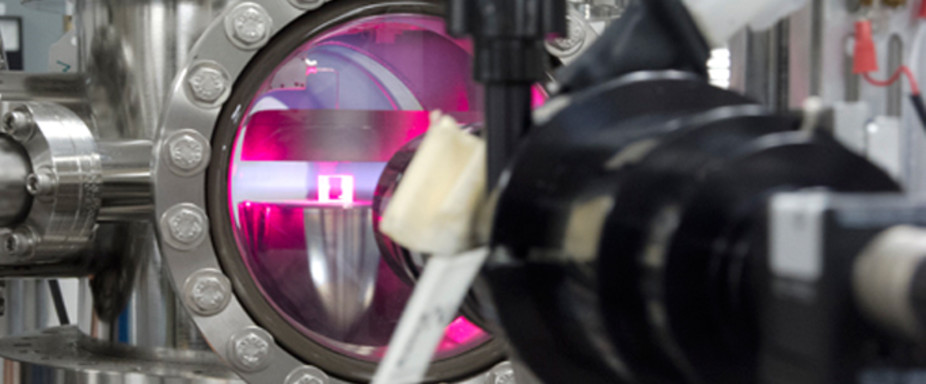
This article was originally published at The Conversation. The publication contributed the article to Space.com's Expert Voices: Op-Ed & Insights.
Augusto Carballido, Baylor University; Jorge Carmona-Reyes, Baylor University, and Truell Hyde, Baylor University
In 1981, the Voyager 2 space probe took intriguing images of Saturn’s rings as it passed by the giant planet on its way to Uranus.
The images showed what looked like ghostly “spokes” moving on the rings, much like the spokes of a bicycle wheel as it turns. After some head-scratching, scientists realized that the Saturnian spokes most likely were tiny specks of dust moving around the rings due to electric and magnetic forces. These forces result from electrically charged gases known as plasma.
Even though you might not realize it based on our daily experiences, researchers think that 99 percent of the matter in our universe is in a plasma state. Many processes that occur in the universe are due in part to the presence of plasma.
Here on Earth, industry uses techniques that involve manipulating these ionized gases for microchip production, as well as for welding materials such as aluminum. Research facilities study plasma in hope of hitting on an efficient nuclear fusion process for energy production. In all these instances micron-size dust is present – which can be a good or a bad thing, depending.
The presence of dust in plasma makes it tricky to study them in a laboratory setting. Here at Baylor University’s Center for Astrophysics, Space Physics and Engineering Research (CASPER), we’ve built a sophisticated experimental facility to investigate these dusty plasma systems. We hope to answer fundamental questions related to dust-plasma interactions both on Earth and in space, in the process advancing our understanding of physics and astrophysics. Our investigations will allow us to untangle issues like the role of dust buildup in super-high-speed crashes in space, the formation of planets, and even areas belonging to life sciences, such as double-helix molecular interaction.
Get the Space.com Newsletter
Breaking space news, the latest updates on rocket launches, skywatching events and more!
Solid swimmers in an electrical sea
Dusty plasma, also called complex plasma, contains small, solid particles distributed throughout the ionized gas. These particles can have the shape of a sphere, a rod or an irregular “pancake.” The reason dusty plasma are very interesting, and even technologically valuable, comes down to the fact that the dust particles themselves can become electrically charged.
We can think of dust particles as swimmers in a sea of electrons and ions – negative and positive charges, respectively. Electrons are so tiny compared to the dust that they easily stick to the particles' surfaces. When that happens, a dust particle will become negatively charged.
Because like charges repel each other, that bit of dust will now feel a push from other negatively charged dust particles. Likewise, that same negatively charged dust particle will feel a pull by the positive ions in the plasma, because charges of opposite signs attract each other. The net result is that dust particles will be pushed and pulled in many different directions, making them move in complex and fascinating ways.
Dusty plasma ‘in the wild’
One place to find dusty plasma is in Earth’s atmosphere, where they’re generated when meteoroids enter the atmosphere’s upper layers at speeds of several kilometers per second. Researchers study them with specialized radars that can detect the ball of plasma that forms around the meteroids as they heat up due to friction with the air.
At high geographic latitudes (between 50° and 70° north and south of the equator) an intriguing phenomenon occurs. Clouds made of tiny ice crystals and dust appear at an altitude of about 80 kilometers during the summer months. Known as noctilucent clouds, they are too thin to be visible in daylight, but they glow before sunrise or after sunset when the sun is below the horizon.
The clouds' icy dust particles are thought to play a role in the chemical composition of the atmosphere at those altitudes. In particular, their presence may be related to depletion of sodium and potassium. And since noctilucent clouds reside in an ionized region of the atmosphere (specifically, the lower ionosphere), their icy dust particles become electrically charged by electrons. These conditions give rise to a variety of electromagnetic phenomena researchers are actively investigating.
Farther from home, but still within our planetary neighborhood, comets can put on an amazing show thanks to dusty plasma effects. As a comet approaches the sun, the sun’s heat transforms part of the comet’s ice into vapor. This vapor, which contains many tiny dust grains, engulfs the comet nucleus to form a “coma.” The solar wind, which is a plasma made mostly of electrons and protons, pushes the cometary coma away from the sun and forms the spectacular tail with which we are familiar.
Even farther from us still, at distances of hundreds of light-years, dust particles embedded in huge, donut-shaped gas clouds move in orbits around young stars. These disks, known as protoplanetary disks, are where planets are formed. Portions of protoplanetary disks may be electrically charged and perhaps threaded by magnetic fields. The dust grains that have been detected in many of these disks are small enough to soak up plasma electrons, and it’s likely that grain charging affects how easily grains can grow to form planet precursors.
Astronomers detect these dusty plasma in space by capturing the light dust particles re-emit after being illuminated by stars. It requires extremely sophisticated instruments, such as the Hubble Space Telescope. From these observations, one can infer things like the dust size and chemical composition.
Dusty plasma in the lab
From a long distance, we can obtain only a limited amount of information about natural dusty plasma. By carrying out controlled experiments in laboratories, we can learn more about dusty plasma properties. For example, by directly manipulating the amount of ionization, we can control the arrangement of the dust particles. In contrast, at this time it is generally not possible to infer the precise arrangement of dust particles in space from telescopic observations.
To experimentally study dusty plasma, a lab must be capable of producing, confining and manipulating an ionized gas – that is, one that contains electrically charged particles. This is possible here at Baylor’s CASPER, where we use advanced instrumentation to investigate the behavior of dusty plasma. For example, we use an instrument called an inductively driven plasma generator – basically a wind tunnel containing electrically charged gases – to study a plasma’s interaction with lunar dust.
We’re also interested in what happens in the plasma when very fast dust particles collide with different targets. Understanding more about these collisions could help with designing better protection for spacecraft from micrometeorite impacts.
CASPER is one of a very few experimental facilities in the world that has three operating complex plasma cells – the chambers into which different gases can be injected to be ionized. Two are Gaseous Electronics Conference radio-frequency reference cells, a standardized plasma-producing chamber. The third is a custom-built device designed here that allows for the formation of much larger complex plasma systems than normally possible. The laboratory within which these cells reside was built from the ground up specifically for them. It’s a highly stable environment with regard to temperature and vibration, making it possible to produce extremely low-noise systems.
A typical experiment looks like this: a glass box can be placed between two electrodes to create an electric potential that will confine the dust-plasma system. The dust particles, which are made of melamine formaldehyde and have a diameter of less than 9 micrometers (about a thousandth of a centimeter in size), are introduced into the box through a dust shaker. Once the system power is turned on, the electric forces will change the arrangement of the dust particles, which will form linear or helical chains, depending on the amount of power used. Images of the dust structures can be captured by telescopic cameras.
These experiments open a window into the way the universe works, whether we are looking for improved efficiency of industrial processes or trying to understand how our solar system formed.
Augusto Carballido, Assistant Research Professor, Baylor University; Jorge Carmona-Reyes, Assistant Director of Educational Outreach and Research, Center for Astrophysics, Space Physics, and Engineering Research , Baylor University, and Truell Hyde, Professor of Physics and Vice Provost of Research, Baylor University
Join our Space Forums to keep talking space on the latest missions, night sky and more! And if you have a news tip, correction or comment, let us know at: community@space.com.