Hunt Continues for Gravitational Waves from Black Hole Megamergers
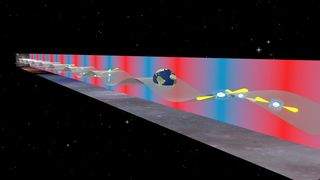
The sound of merging supermassive black holes does not saturate the universe.
For the past decade, scientists with the North American Nanohertz Observatory for Gravitational Waves (NANOGrav) collaboration have been listening for a constant "hum" of low-frequency gravitational waves.
Theoretical work suggests that this hum — generated by collisions involving supermassive black holes, which contain millions or billions of times more mass than the sun — should be detectable at Earth. NANOGrav hasn't heard the hum yet, a new study reveals, but this lack of detection is an interesting result in its own right, revealing new details of how galaxies might evolve and merge, team members said. [The Search for Gravitational Waves in Pictures]
Galactic gravitational wave detectors
Gravitational waves — cosmic ripples that distort the fabric of space-time itself — were first predicted by Albert Einstein in his theory of general relativity in 1916.
The first direct detection of these ripples was announced earlier this year, by researchers with the Laser Interferometer Gravitational-Wave Observatory (LIGO) project. The waves spotted by LIGO radiated outward from a cosmic crash between two black holes that were 29 and 36 times the mass of the sun, respectively.
The $1 billion LIGO experiment bounces a laser beam between mirrors that are positioned at either end of two 2.5-mile-long (4 kilometers) arms. If a gravitational wave passes through the detector, the length of one of these arms will change ever so slightly compared to the other, affecting the arrival time of the laser light by a fraction of a second.
NANOGrav works on the same general principle, but it uses pulsars — dead stars that spin rapidly, up to hundreds of times each second — instead of Earth-based laser beams. And it hunts for supermassive black holes.
Get the Space.com Newsletter
Breaking space news, the latest updates on rocket launches, skywatching events and more!
Pulsars emit beams of radiation from their magnetic north and south poles. If a pulsar happens to be aligned with Earth just right, astronomers will see flashes of its radiation, just as observers can see beacons of light from a lighthouse. These flashes can contain evidence of gravitational waves.
"As the gravitational wave transits the Milky Way, it stretches and squashes space-time, making the pulsars and the Earth jiggle," Chiara Mingarelli, an astronomer from the California Institute of Technology in Pasadena, told Space.com. "And we can measure that jiggle by looking at changes in the arrival time in the pulses."
Two pulsars "are like the interferometer arms on LIGO, but instead of being 4 kilometers long, they're 3,000 light-years long,” Mingarelli added. "A pulsar timing array is really a galactic-scale gravitational-wave detector."
NANOGrav currently monitors 54 pulsars throughout the Milky Way using the Green Bank Telescope in West Virginia and the Arecibo Observatory in Puerto Rico, the two most sensitive radio telescopes operating at the necessary frequencies. Team members are hoping to hear a distinctive low-frequency sound.
"Whereas LIGO has this high-pitched chirp, pulsar timing arrays have a low growl from all these supermassive black holes combined," Mingarelli said. [What Colliding Black Holes Sound Like (Video)]
Why the silence?
When two galaxies collide, the supermassive black holes at the cores of each sink to the center of the newly created megagalaxy and swirl around one another. Scientists think that, over time, the black holes' orbits shrink, allowing them to merge and form an even more gigantic supermassive black hole.
But there's a problem with this scenario. While it’s easy to imagine how two supermassive black holes could come to orbit one another, astronomers struggle to model how the behemoths cross the final parsec (about 3.2 light-years) of space between each other to merge. In order to fall toward each other, the black holes have to radiate away energy — but exactly what that energy looks like, and how it changes as the black holes come closer together, is relatively unknown.
The simplest model predicts that black holes merge via gravitational-wave radiation for that entire final parsec. If that's the case, the supermassive black holes would radiate so many gravitational waves that a background "hum" should have been detected by now. But the new analysis of NANOGrav's nine-year dataset did not detect any such background.
However, that result isn’t surprising, said study co-author Justin Ellis, an astronomer at NASA's Jet Propulsion Laboratory in Pasadena.
"Most people in the field didn't really believe the optimistic model," Ellis told Space.com.
The likelihood that the background noise is relatively weak means that the physics involved are a little messier — and more interesting, researchers said.
There are two possible interpretations of the failure of the NANOGrav collaboration to detect any telltale noise until now. The first is that merging supermassive black holes shrink in their orbits because they interact with stars and gas. If the black holes slingshot stars away from the center of the galaxy, for example, that could cause them to lose the necessary energy and slouch toward the center. This would cause them to merge faster and emit less gravitational-wave radiation.
The other possibility is that the black holes spiral toward each other so slowly — on timescales longer than the age of the universe — that they essentially never merge.
"It’s weird to think that the centers of galaxies just have these massive binaries that just can't get together, but it's possible," Mingarelli said.
Within these models are many knobs that theorists can turn, Ellis said. Researchers can play with how quickly and efficiently supermassive black holes merge via interacting with gas and dust, slingshotting stars away from the center or simply emitting gravitational-wave radiation.
"It's a big parameter space, and this is the first study that can start to cut out some of the edges of that parameter space and limit how far the knobs can be turned," Ellis said.
The new study was published in The Astrophysical Journal.
Follow Shannon Hall on Twitter @ShannonWHall. Follow us @Spacedotcom, Facebook or Google+. Originally published on Space.com.
Join our Space Forums to keep talking space on the latest missions, night sky and more! And if you have a news tip, correction or comment, let us know at: community@space.com.
Shannon Hall is an award-winning freelance science journalist, who specializes in writing about astronomy, geology and the environment. Her work has appeared in The New York Times, Scientific American, National Geographic, Nature, Quanta and elsewhere. A constant nomad, she has lived in a Buddhist temple in Thailand, slept under the stars in the Sahara and reported several stories aboard an icebreaker near the North Pole.