Collapse or Collision: The Big Question in Star Formation
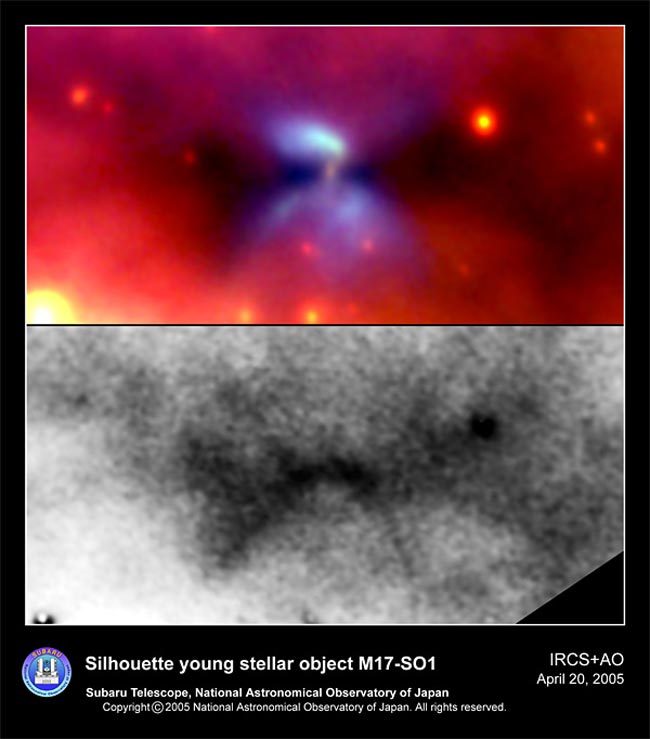
A young star that had been the poster child of massive star formation may have been lying about its weight.
The star is in its infant stages and is known as a protostar, with the designation M17-SO1. Its heft carries weight in an ongoing debate over how the most massive stars form.
A year ago, a group of astronomers published evidence showing that the mass of M17-SO1 was more than 15 times that of our Sun. The radiation from a star this big is thought to prevent matter from collapsing onto it. But if matter cannot fall - or accrete - onto a massive star, how does it grow to be so large?
"It's the big question in star formation at this moment," said Markus Nielbock of the Ruhr-Universitat Bochum in Germany, who along with Rolf Chini measured the mass of M17-SO1.
One theory states that stellar behemoths are built out of the collision of two medium-sized stars. But Chini and Nielbock found signs that M17-SO1 was chomping away on an accretion disk, which implies the same kind of slow feeding process that goes on in smaller stars.
"[Chini's group] proposed that high mass stars evolve in a similar manner to low/mid mass stars by going through a phase with a flared disk," said Shigeyuki Sako from the University of Tokyo. "This was a shock to the community studying the formation of high mass stars, especially to the theorists."
From their own data for M17-SO1, Sako and his colleagues claim the star has less than 8 solar masses, meaning radiation pressure is no longer a concern. Whether this is good news or bad news depends on how one thinks massive stars come into being.
Get the Space.com Newsletter
Breaking space news, the latest updates on rocket launches, skywatching events and more!
Dust buster
The mass of M17-SO1 cannot be determined directly because the star is embedded inside the Omega Nebula in the constellation Sagittarius. In optical light, there is almost nothing to see, so astronomers use infrared instruments, like the Subaru telescope, to penetrate the dust.
"Determining the mass of a star surrounded by proto-stellar/proto-planetary disk is difficult since the surrounding material obscure the central star, especially when we are looking at the system edge-on," Sako said in an email message.
The edge-on view of M17-SO1 shows a butterfly-shaped envelope, 150 times the size of our solar system. Sako's group was able to map out some of the structure.
"The new observations show a circumstellar disk - the cradle of planets - in the process of forming," Sako said.
Some of the gas in the envelope is noticeably swirling around the star. By measuring the rotation speed of this gas with radio observations, astronomers can try to estimate the mass in the center.
In this way, Chini's group determined that M17-SO1 is 15 to 20 times the mass of our Sun and claimed that this agreed with how bright they estimated the star to be. But the recent work of Sako's team, reported in the 21 April issue of Nature, give a mass between 2.5 and 8 solar masses.
According to Sako, analyzing rotation velocities requires assumptions about the temperature and composition of the gas. This leads to large uncertainties in the mass estimate. Chini said they have new data - not yet fully compiled - that may resolve the issue.
Ionizing power
But to support their lower mass estimate, Sako and company argue that M17-SO1 cannot be greater than 8 solar masses because, otherwise, its radiation would ionize the hydrogen gas in its neighborhood.
Although ionized hydrogen is not seen around M17-SO1, Chini said that this only implies that the star is very young, and therefore, it is too soon to see signs of stellar ionization.
Beyond this debate, though, the onset of ionizing radiation is one of the reasons that stars greater than 8 solar masses are thought to be so hard to form.
Small and medium sized stars are born through a collapse of gas, followed by a gradual accretion of matter through a stellar disk. But once a star reaches about 8 solar masses, its radiation is thought to blow away any remaining material - cutting off the gas supply.
Coalescence
The alternative to collapsing accretion is that stars in dense clusters collide and coalesce to form bigger stars. This would avoid any kind of radiation cutoff, but there is not much evidence that this scenario can work.
"The problem with the collision theory is that there is no observational tool to measure, i.e. to prove that such a collision has occurred," Chini said.
Chini also said that computer models have improved over the years, and it is no longer so hard to model an accretion history for a very massive star.
"Why should nature invent two mechanisms for the same process?" Chini asked. "[Accretion] works perfectly from 0.1 to 10 solar masses and - as theorists show - also beyond."
Hard to catch
But if Sako's group is correct, Chini and others will have to look for another massive star feeding on an accretion disk. These are rare birds in the field of astronomy.
"The problem is we almost never get to see a young massive star because they form so quickly," said Debra Shepherd of the National Radio Astronomy Observatory, who was not involved with either group.
Shepherd explained that if massive stars form through accretion, they gain about a solar mass every 1000 years, so they're finished growing in as little as 20,000 years.
"That's just a blink of an eye," she said.
- How Magnetic Fields Help Stars Form
- How a Star is Born: Clouds Lift on Missing Link
Join our Space Forums to keep talking space on the latest missions, night sky and more! And if you have a news tip, correction or comment, let us know at: community@space.com.
Michael Schirber is a freelance writer based in Lyons, France who began writing for Space.com and Live Science in 2004 . He's covered a wide range of topics for Space.com and Live Science, from the origin of life to the physics of NASCAR driving. He also authored a long series of articles about environmental technology. Michael earned a Ph.D. in astrophysics from Ohio State University while studying quasars and the ultraviolet background. Over the years, Michael has also written for Science, Physics World, and New Scientist, most recently as a corresponding editor for Physics.