Dark energy is forcing the universe to expand. This new observatory may show us how
The Rubin Observatory is set to achieve first light next year. It could finally reveal some secrets about the dark universe.
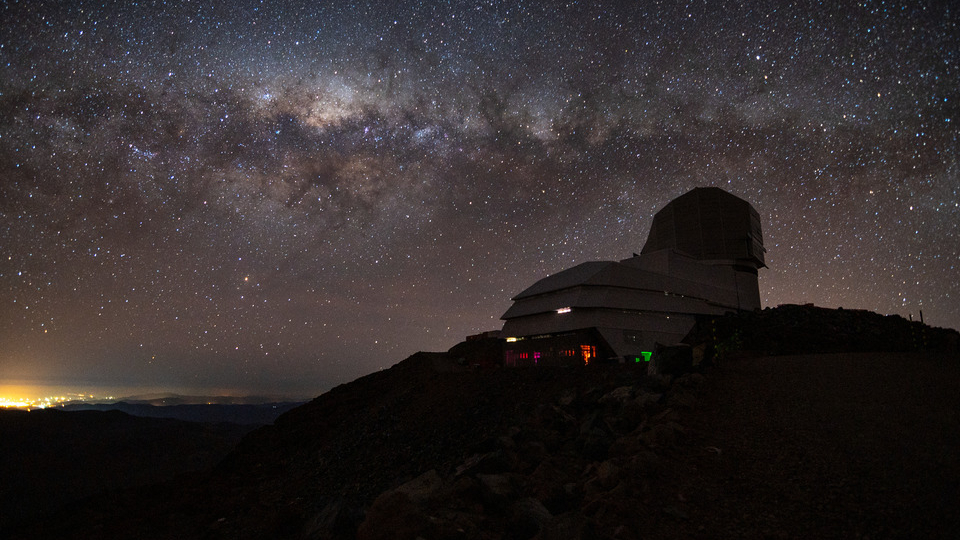
As our universe poofs out in every direction like an indestructible balloon — thanks to dark energy, a force fully hidden to the human eye — Dillon Brout is an astrophysicist trying to make sense of it all.
Brout wishes to unveil the strange correlation that exists between the invisible and visible universe, understand how the fabric of spacetime flows and perhaps finally reveal the truth about whatever's causing the cosmos to bubble outward faster and faster by the day.
To do this, he collects supernovas.
When picking out which supernovas to add to the shelf, however, Brout isn't interested in them all. These star explosions are typically divided into two main categories: Type 2 and Type 1a. Brout wants the Type 1a's, and his reasoning is actually pretty simple:
"They're not all exactly the same, but they're very similar," he told Space.com.
Related: How fast is the universe expanding? New supernova data could help nail it down
In essence, to solve all those aforementioned space mysteries, you need to measure some distances on cosmological scales. Only then can you know, for instance, how far and how quickly dark energy seems to have forced space to expand. Reverse calculate from there, and maybe you learn something about the nature of dark energy itself, too. Yet, to measure even such grand distances and elusive concepts, to probe how far back we can see and how much farther back that point is traveling, you still need something as basic as a ruler.
Get the Space.com Newsletter
Breaking space news, the latest updates on rocket launches, skywatching events and more!
Fortunately, because they're so standardized in brightness and general behavior, Type 1a supernovas are like the ticks on light-years-long rulers plunging through space. In fact, astronomers like to call them "standard candles" for this reason. They're perfect lighthouses that guide us as we calibrate our equations and search for some answers. The more we have, the better.
Looking for efficiency and accuracy, Brout fills up his supernova collection by employing machine learning algorithms that vigorously scout out as many Type 1a's as possible. (Yet another reason why Type 1a standardization is helpful. Consistent algorithms love consistency.)
He's part of the Dark Energy Survey collaboration, and earlier this month, the team announced their algorithms managed to detect 1,500 of these luminous natural markers — in only five years. That's a pretty big deal. For context, Brout says it took scientists 30 years of regular Type 1a searching (aka, through using a trusty spectrograph) to find the previous 1,500 total subjects. DES got the same result within about a sixth of that timeframe.
"One of the main things that made DES so special is that it covered so much area on the sky," Brout said, adding that he trusts his algorithms enough to say cross-checking the same parameters is more or less not needed.
But things are about to heavily ramp up.
Though DES yielded an impressive amount of Type 1a's, its associated instrument, the Dark Energy Camera, only covered 30 square degrees of sky. That's a relatively small fraction, Brout says. Enter: The Rubin Observatory.
Or, more specifically, the Legacy Survey of Space and Time that'll be created in part by using the state-of-the-art LSST Camera starting next year.
"LSST is going to observe the entire observable southern night sky," Brout said. "You're going to go from DES discovering 1,500 to LSST discovering a million alerts, and we're going to filter that down, hopefully, using machine learning and other algorithms to get a few 100,000 Type 1a supernovas."
One specific question waiting to be answered
Fortunately once more, the Rubin Observatory is officially on track to be totally built later this year and the LSST will begin its journey early-to-mid next from the top of a Chilean summit, Victor Krabbendam, the observatory's construction project manager said during the 243rd meeting of the American Astronomical Society in January 2024. "We're about 10 years into the actual construction phase," he said. "The sun is setting and we're getting close."
And actually, Brout already has a specific puzzle waiting to be solved with the LSST.
With their major 1,500 Type 1a supernova haul announced this month, Brout and fellow researchers sort of confirmed what we presently know about what's called "the cosmological constant," which you can think of as the value that represents dark energy in the universe's expansion equations. It accounts for the acceleration bit that normal physics can't totally explain. This "confirmation" might sound disappointing at first, but in a way, it's quite good progress. It means that one of the most precise calculations of the universe's expansion is telling us that we're probably right about everything we know concerning dark energy so far.
Maybe more interesting, however, is that the team's work sort of hinted at a weird pattern, too. "We do have a section in the paper that combines all of the available probes of dark energy, not just supernova, and what we see is a lot of them are pointing towards a slightly larger value of the 'equation of state' of dark energy, which would imply that it's not a cosmological constant."
In other words, that'd mean there isn't a value to blanket represent dark energy. Maybe it's flexible.
"One of the major benefits we get from this new LSST analysis is that we get a lot more supernovas in the nearby universe, and that's because we're covering so much area of the sky," Brout said. "If you think about it, the nearby universe is the universe that, because of the speed of light, we're seeing the galaxies much closer to as they are today. If you're looking at the faraway universe you're seeing the universe when it was much younger."
That's important, he explains, because the effect of dark energy is believed to be strongest in the recent universe. Why? Here's where it gets really weird.
"Dark energy, we think, is a property of space itself," Brout said. "That's kind of what the cosmological constant embodies, which is like the energy of empty space."
Thus, if dark energy is a property of empty space, that'd mean there's more dark energy in the universe today than there was in the past. This is because the universe is expanding, thereby creating more "space."
"We think it does not dilute as the universe expands," Brout said, "so that means, relative to the amount of matter in the universe and dark matter in the universe, you're getting more and more dark energy."
At this point, like I was, you might be wondering: I'm sorry, what? I thought the universe is contained? Where is the new dark energy coming from? It can't just pop into existence, right?
"That's the million dollar question," Brout said. "Is it just a property of space? Is this a fundamental property of the universe? That as space itself expands, you would just naturally get more dark energy along with it?"
And to get to the bottom of this, we'll soon have a multi-million dollar camera waiting.
2025's golden observatory
There are four major steps left before Brout can start counting the days leading up to LSST's first light. First, the Rubin team must get some key mirrors ready to go. Then, the crew must get the glass necessary for the Simonyi Telescope — which reportedly has flown through tests without even the proper glass component — and mount the commissioning camera thereafter. Finally, the approximately $200 million LSST camera, currently being put together on the West Coast, will earn its spot.
"You still have to get that from California to the summit. It's a very delicate instrument. It's special in the sense that it's a $200 million camera — irreplaceable," Krabbendam told Space.com.
"It is a massive camera," he said. "It's 3.2 gigapixels for a focal plane."
One gigapixel, for context, is equal to one billion pixels; a standard DSLR camera works on scales of megapixels, or millions of pixels. To really drive this home, consider how a million seconds is 12 days; a billion seconds is 31 years. So… picture that resolution of camera power scanning the entire observable southern sky.
This is why the observatory, built with about $500 million of National Science Foundation funding and a few $100 million of Department of Energy funding — the latter of which is particularly interested in dark energy studies like Brout's — is so highly anticipated.
So highly anticipated.
Update 2/1: 1 million seconds is equal to 12 days, this article has been updated to reflect that.
Join our Space Forums to keep talking space on the latest missions, night sky and more! And if you have a news tip, correction or comment, let us know at: community@space.com.
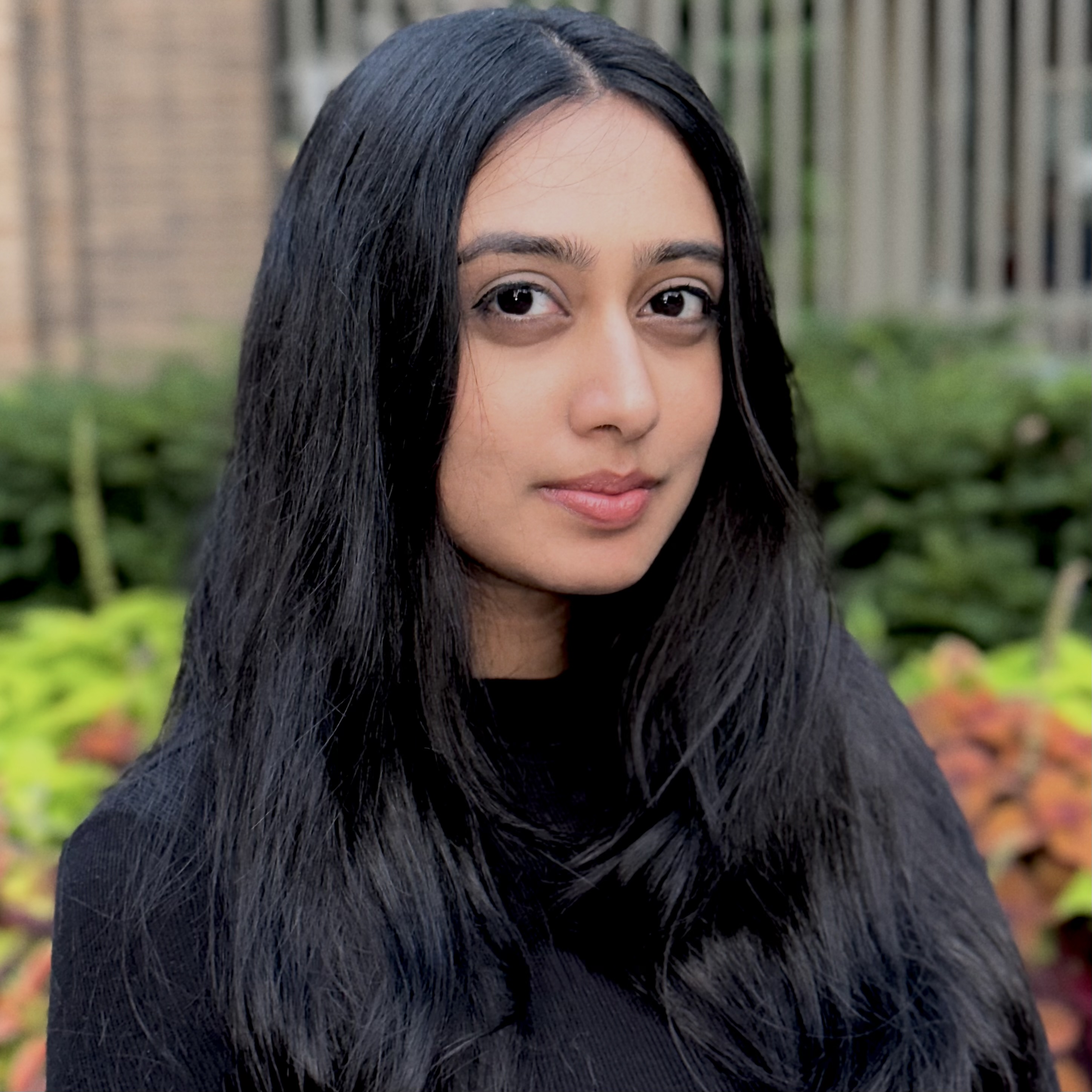
Monisha Ravisetti is Space.com's Astronomy Editor. She covers black holes, star explosions, gravitational waves, exoplanet discoveries and other enigmas hidden across the fabric of space and time. Previously, she was a science writer at CNET, and before that, reported for The Academic Times. Prior to becoming a writer, she was an immunology researcher at Weill Cornell Medical Center in New York. She graduated from New York University in 2018 with a B.A. in philosophy, physics and chemistry. She spends too much time playing online chess. Her favorite planet is Earth.
-
Ed Stauffer If dark matter changes states between a liquid and gaseous state then there would have been a time where almost all of the dark matter cooled, condensed and collapsed. This liquid state contraction could possibly have led to direct collapse black holes and galaxies. The condensing of dark matter may have also contributed to that uniformity of temperature.Reply
If the dark matter was in its liquid state then baryonic and dark matter would have been much more concentrated. This would have resulted in deeper gravity wells. The time in these gravity wells to us would seem to be moving slower to us. But due to dark matter condensing the baryonic matter would also have been cooled and rushing together.
Once stars were formed and black holes became active the ratio of liquid to gaseous dark matter would have decreased over time thus affecting the evolution of particle masses. And making the gravity wells progressively shallower and larger in diameter over time. Galaxy clusters would have evaporated almost all of their liquid dark matter resulting in the shallowest part of the gravity well being near the canter of the cluster. Also part of redshift is due to the difference depth of the average gravity well at that point in time compared to now. The slope of that line would also have decreased over time.
Light red shifts as it climbs out of a gravity well. Thus the further you go back in time the more light is redshifted. This would leave everything the same with the exception of our perception that the universe is expanding. Also if a big portion of the redshift is from climbing out of a deeper gravity well then we are not looking as far into the past as we think. -
Questioner I will venture a guess that DE is higher proximate to visible matter.Reply
That it isn't a property of space itself, but arises from activity/events of visible matter.
Side note: quite possibly DE is combined from more than one physics source.