Nuclear fusion reactor 'breakthrough' is significant, but light-years away from being useful
Useful, cost-effective nuclear fusion remains a distant dream, despite a small step in the right direction from the government's NIF reactor.
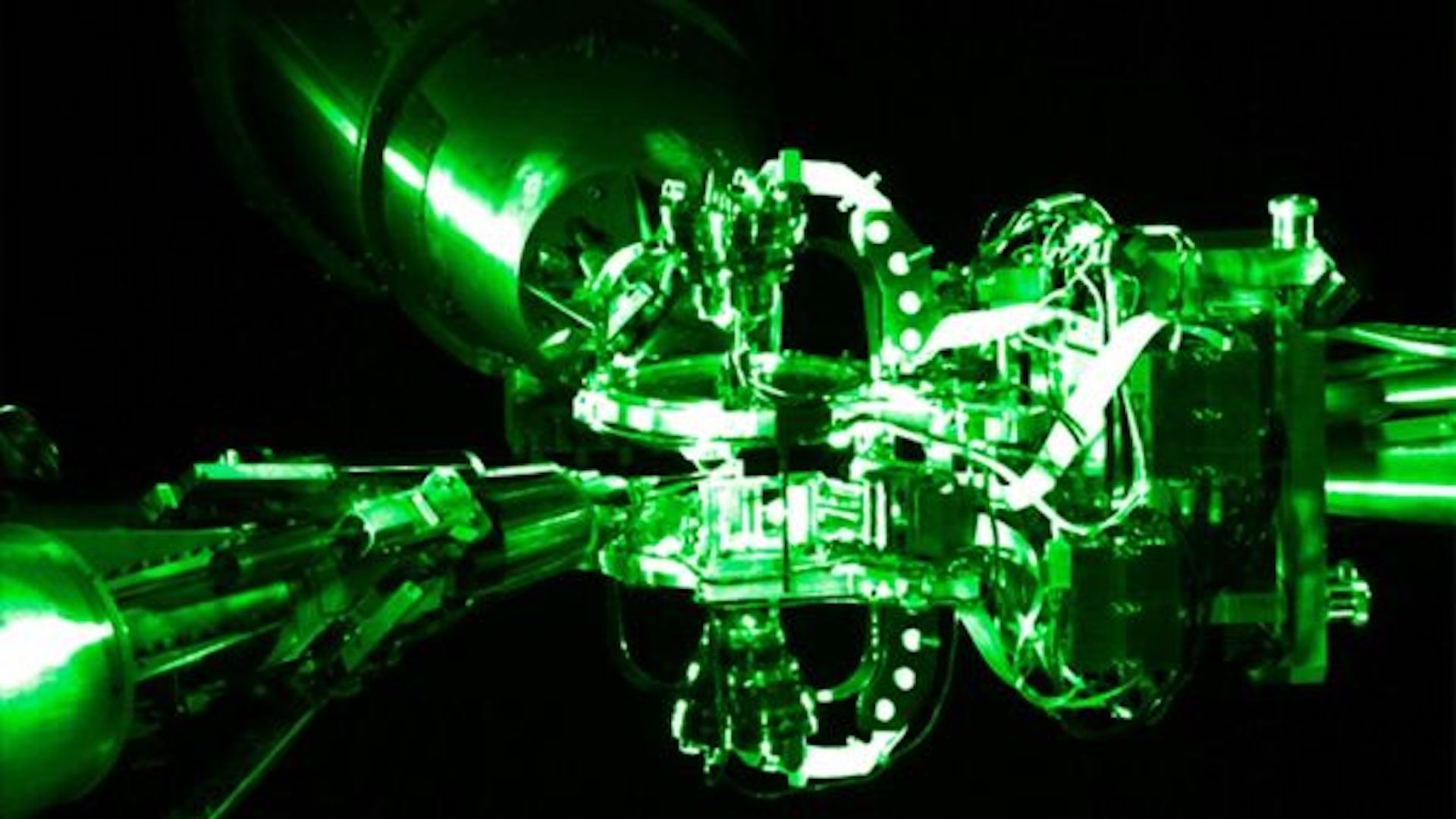
Scientists have just announced a breakthrough in nuclear fusion ignition: For the first time the heart of a powerful fusion reactor has briefly generated more energy than was put into it. But experts are urging caution, saying that the breakthrough, while hugely significant, is still a long way from safe, limitless nuclear energy.
On Tuesday (Dec. 13), physicists at the U.S. government-funded National Ignition Facility (NIF) at Lawrence Livermore National Laboratory in California announced that they were able to fire a laser carrying roughly 2 megajoules of energy into a tiny fuel pellet made up of two hydrogen isotopes, turning the atoms into plasma and producing 3 megajoules of energy — a 50% increase.
Scientists are very excited by the results, but wary of overhyping them. The reactor as a whole did not produce a net gain of energy. For a fusion reaction to be practically useful, the tens of megajoules drawn from the electrical grid, converted into the laser beams and fired into the reactor core would have to be significantly less than the energy released from the plasma.
Related: What is nuclear fusion?
But the new plasma ignition milestone only accounts for the laser energy in and the plasma energy out, not the sizable loss from converting electricity to light.
What's more, the reaction takes place in a tiny fuel pellet inside the world's biggest laser, lasts only a few billionths of a second, and can only be repeated every six hours. This makes the reaction far too inefficient for practical purposes.
"Net energy gain is a significant milestone, but to put it in perspective, it means fusion is now where Fermi put fission about eighty years ago," Ian Lowe, a physicist and emeritus professor at Griffith University in Australia, told Live Science. "The huge technical problem is maintaining a mass of plasma at a temperature of several million degrees to enable fusion, while extracting enough heat to provide useful energy. I still haven't seen a credible schematic diagram of a fusion reactor that achieves that goal."
Get the Space.com Newsletter
Breaking space news, the latest updates on rocket launches, skywatching events and more!
Read more: Nuclear fusion breakthrough: What does it mean for space exploration?
How fusion reactors work
Existing fusion reactors can be split into two broad categories: inertial confinement reactors like the NIF's, which contain the hot plasma with lasers or particle beams, and magnetic confinement reactors, such as the U.K.-based Joint European Torus (JET), Europe's upcoming International Thermonuclear Experimental Reactor (ITER), and China's Experimental Advanced Superconducting Tokamak (EAST), which sculpt the plasma into various torus shapes with strong magnetic fields. At ITER, the field confining the burning plasma will be 280,000 times as strong as the one around Earth.
The varying reactor types reflect different strategies for overcoming fusion's intimidating technical barriers. Magnetic confinement reactors, known as tokamaks, aim to keep the plasma continuously burning for prolonged periods of time (ITER's goal is to do this for up to 400 seconds). But, despite edging ever closer, tokamaks have yet to create a net energy gain from their plasmas.
On the other hand, inertial confinement systems like the NIF reactor, which also operates to test thermonuclear explosions for military purposes, generate bursts of energy by quickly burning one tiny chunk of fuel after another. This fuel, however comes in the form of discrete pellets, and scientists have yet to figure out how to replace them quickly enough to maintain a reaction for longer than the tiniest fraction of a second.
"That is very, very tricky because it would mean that you need to position your next pellet during the time that the [plasma] cloud expands in the vessel," Yves Martin, the deputy director of the Swiss Plasma Center at the École polytechnique fédérale de Lausanne in Switzerland, told Live Science. "This pellet is typically one millimeter [0.04 inches] big in diameter and it has to be positioned in a room which is nine meters [30 feet] across. As far as I know, it still costs several tens of thousands of dollars [to get the reaction going]. To be interesting, it should go down to one dollar or even less."
Read more: Chinese nuclear reactor proposal could power 10 International Space Stations
A very expensive isotope
Another problem for fusion reactors is the dwindling supplies of tritium, a key isotope that is combined with deuterium as fuel for the reaction. Once a common and unwanted byproduct of open air nuclear weapons tests and nuclear fission — which splits atoms instead of combining them and produces far more radioactive waste — tritium's 12.3-year half-life means that much of its existing stock is already on the way to being unusable, making it one of the most expensive substances on Earth at $30,000 per gram.
Physicists have proposed other methods for making tritium, such as breeding it inside nuclear reactors that capture stray neutrons. But, besides some smaller scale experiments, rapidly ballooning costs meant plans to test tritium breeding at ITER had to be scrapped.
Fusion researchers believe that if the political will can be found and the engineering challenges solved, the first viable fusion reactors could come online as soon as 2040. But that's still ten years too late to keep global warming below the target of 1.5 degrees Celsius (2.7 degrees Fahrenheit), by 2030.
"Decision makers yearn for the holy grail of clean energy from an abundant resource," Lowe said. "Having spent squillions on fusion research, they are very reluctant to give up, just as they spent decades chasing the fantasy of the breeder reactor [a fission reactor which outputs more energy than it consumes]."
Nevertheless, recent years have seen improvements to fusion technology arriving in a steady stream. These include a successful trial of AI to control the plasma inside a tokamak; a slew of records in power generation, plasma burn time, and reactor temperatures across multiple experiments; and the rewriting of a foundational rule which could enable future reactors to generate twice as much power. In light of these advances, fusion scientists insist that multiple strategies for a long-term solution to the climate crisis are necessary, and that fusion will become a vital component of a future carbon-free energy system.
"If we wanted to rely on renewables only, we would need such an excess of installations to have the amount of energy you would typically need in winter, or in a period with no wind. We need something which will be the base level that will produce exactly what you want," Martin said. "It's not because I believe in fusion that I will not put some solar panels on my roof. In a sense, we really need to use everything that is better than fossil fuels."
Originally published on LiveScience.com
Join our Space Forums to keep talking space on the latest missions, night sky and more! And if you have a news tip, correction or comment, let us know at: community@space.com.
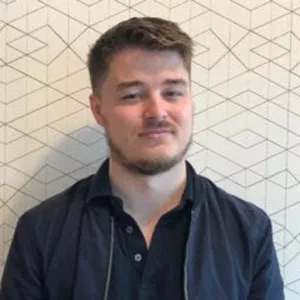
Ben Turner is a U.K. based staff writer at Live Science. He covers physics and astronomy, among other topics like weird animals and climate change. He graduated from University College London with a degree in particle physics before training as a journalist. When he's not writing, Ben enjoys reading literature, playing the guitar and embarrassing himself with chess.
-
Jan Steinman "light-years away"Reply
Don't you just love it when people conflate time with distance?
Or, perhaps they meant that they've discovered a civilization with working fusion energy that is mere "light-years away."
I prefer harnessing the fusion power plant that is about eight light-minutes away. -
ChrisA They use the word "breakthrough" in the headline but fail to report what it is. They say the effect is 50% more energy out then used by the laser but what was the breakthrough? What did they discover to enable this result?Reply
It is horrible science reporting when the science is left out
This is like posting "new design causes car to use 30% less gas" then failing to say what the new design is.
e all know why this was left out, the article that was sumerixed to make this one also left out the data. This is what we get with "copy/pate" journalism. We get flooded with same stuff -
orsobubu
I think cold fusion (lenr, evos, etc) could succeed before the hot fusion utopia; hot fusion, and in particular the livermore experiment, is useful to attract investments for various businesses and academic careers and to advance plasma physics to maintain the thermonuclear arsenal and to develop the future ones.Jan Steinman said:"light-years away"
Don't you just love it when people conflate time with distance?
Or, perhaps they meant that they've discovered a civilization with working fusion energy that is mere "light-years away."
I prefer harnessing the fusion power plant that is about eight light-minutes away. -
Vernon Brechin The report of this so called 'breakthrough' came from people who are well versed in obscuring key details because they work in the secret environment of a nuclear weapons laboratory, Lawerence Livermore National Laboratory (LLNL). Within it is the National Ignition Facility (NIF) giant laser building which has always been primarily funded to study thermonuclear weapon (H-bomb) fuel-compression and other thermonuclear weapon's dynamics. The reported experiment was actually part of that function. Material samples were placed next to the fuel capsule to test their reaction to the intense pulse of 14 Mev fusion neutrons that were generated by the reported experiment.Reply
The reported input energy quantity may not have been from the lasers but instead a measure of the fraction of X-rays that ended up compressing the extremely expensive, nearly perfectly spherical, hollow diamond fuel capsule. The fusion energy production lasted about 0.08 nanoseconds. Since it took about a week to prepare the shot the duty cycle of this shot, over a one-week period, would be around 0.0000000000000002. It was finally admitted by the NIF staff that the energy needed to charge up the lasers was over 100 times greater than that used as the input reference energy to the compress the the fuel capsule. During the exceedingly brief reaction about 4% of the once frozen deuterium/tritium (D/T) fuel mixture actually 'burned' with the remaining ending up as radioactive waste coating the interior of the vacuum vessel that required cleaning out before another experiment could be performed.
The lab employs their own definition of 'ignition' that is different than most people idea of ignition. Most people equate it with something like a match head striking a rough surface where the initial reaction spreads to the rest of the fuel in about two seconds. In the NIF inertial confinement experiment (ICF) the extremely compressed core of the fuel pellet, which was in the form of a 150 million kelvin plasma, started the fusion reaction then blew off the other 96% of the fuel before it could participate in the reaction. The fusion reaction is totally dependent upon the energy contained within the laser beams and the compressing X-rays that are generated from those beams. During the blast from the laser beams the entire expensive target assembly turns into an intensely hot plasma.
The multibillion dollar National Ignition Facility (NIF) lasers achieved full power in 2009 and the experimenters were expected to achieve its namesake goal of 'ignition' by 2012. It failed by a wide margin but the funding kept flowing from the U.S. nuclear weapons program budget.
I've followed the fusion energy experiments since the early 1970s with its usual claims that a practical fusion power reactor will likely only be 20-30 years away, touted by the experimenters and their fans. They often state that the power source is virtually unlimited since the fuel can be obtained from seawater. The fact is that tritium is extremely rare, extremely expensive and radioactive, having a half-life of about 12 years. There are enormous problems needed to be overcome for future fusion reactors to breed their own tritium fuel from a lithium isotope. The intense energy 14 Mev neutrons, generated by the fusion reactions, will degrade the structures around them and will render some of their atoms radioactive via the process of neutron activation reactions. The usual claims of fusion producing clean energy without creating radioactive waste is pure deceptive sales hype. Most nuclear fusion physicists agree that Magnetic Confinement Fusion (MCF) experimental approaches are far more likely to lead to a practical fusion power plant design. Still that is likely to take well over a single decade. Scaling that up to displace our addiction to fossil fuel based energy resources will take more decades. Those people, who continue to believe that nuclear power will save us, have to be masterful at excluding the following warnings from their consciousness.
IPCC report: ‘now or never’ if world is to stave off climate disaster
https://www.theguardian.com/environment/2022/apr/04/ipcc-report-now-or-never-if-world-stave-off-climate-disaster
UN chief: World has less than 2 years to avoid 'runaway climate change'
https://thehill.com/policy/energy-environment/406291-un-chief-the-world-has-less-than-2-years-to-avoid-runaway-climate * This statement was made 4-years ago.
Here is another critical review of the recent experiment and the ITER situation. When most journalists look for critical balance in their articles, on topics such as this, they tend to defer to those experts who have vested interest in the technology, or have long been fans of it.
Is This the ‘Kitty Hawk Moment’ for Fusion Energy?
https://www.theatlantic.com/technology/archive/2022/12/department-of-energy-nuclear-fusion-breakthrough-nif-livermore/672439
ITER is a showcase … for the drawbacks of fusion energy
https://thebulletin.org/2018/02/iter-is-a-showcase-for-the-drawbacks-of-fusion-energy
ITER Newsline
KEY COMPONENTS TO BE REPAIRED
21 Nov. 2022
https://www.iter.org/newsline/-/3818