Early Universe 'Soup' Cooked Up in Weird Plasma Blobs
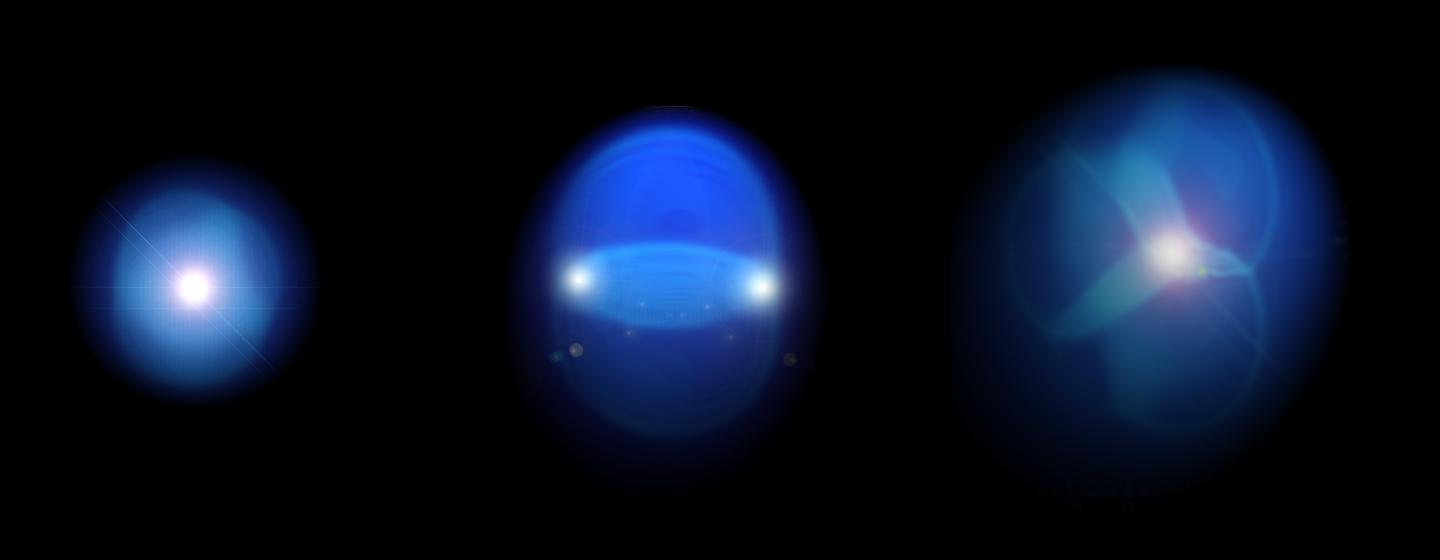
For the first split second after the Big Bang, the universe was nothing but an extremely hot "soup" of quarks and gluons — subatomic particles that would become the building blocks of protons and neutrons. Now, 13.8 billion years later, scientists have re-created this primordial soup in a laboratory.
Using the Relativistic Heavy Ion Collider at Brookhaven National Laboratory in Upton, New York, physicists generated tiny drops of this quark-gluon plasma by smashing together different combinations of protons and neutrons. During these crashes, the quarks and gluons that made up the protons and neutrons broke free and behaved as a liquid, the researchers found.
Depending on which combination of particles the researchers smashed together, the tiny, liquid-like globs of plasma formed one of three distinct geometric shapes: circles, ellipses or triangles. [Images: Peering Back to the Big Bang & Early Universe]
"Our experimental result has brought us much closer to answering the question of what is the smallest amount of early universe matter that can exist," Jamie Nagle, a physicist at the University of Colorado Boulder who participated in the study, said in a statement.
Quark-gluon plasmas were first created at Brookhaven in 2000, when researchers smashed together the nuclei of gold atoms. Then, scientists at the Large Hadron Collider in Geneva defied expectations when they created the plasma by smashing two protons together. "That was surprising because most scientists assumed that lone protons could not deliver enough energy to make anything that could flow like a fluid," UC Boulder officials said in the statement.
Nagle and his colleagues decided to test the fluid properties of this exotic state of matter by creating tiny globs of it. If the plasma truly behaves like a liquid, the little globs should be able to hold their shape, the researchers predicted.
Get the Space.com Newsletter
Breaking space news, the latest updates on rocket launches, skywatching events and more!
"Imagine that you have two droplets that are expanding into a vacuum," Nagle said. "If the two droplets are really close together, then as they're expanding out, they run into each other and push against each other, and that's what creates this pattern."
"In other words, if you toss two stones into a pond close together, the ripples from those impacts will flow into each other, forming a pattern that resembles an ellipse," UC Boulder officials said. "The same could be true if you smashed a proton-neutron pair, called a deuteron, into something bigger ... Likewise, a proton-proton-neutron trio, also known as a helium-3 atom, might expand out into something akin to a triangle."
By ramming these different combinations of protons and neutrons into gold atoms at close to the speed of light, the researchers were able to do exactly what they hoped: create elliptic and triangular blobs of quark-gluon plasma. When the scientists smashed a single proton into the gold atom, the result was a circular blob of the primordial soup.
These short-lived droplets of quark-gluon plasma reached temperatures of trillions of degrees Celsius. Researchers think that studying this type of matter "could help theorists better understand how the universe's original quark-gluon plasma cooled over milliseconds, giving birth to the first atoms in existence," UC Boulder officials said.
The results of this study were published Dec. 10 in the journal Nature Physics.
Email Hanneke Weitering at hweitering@space.com or follow her @hannekescience. Follow us on Twitter @Spacedotcom and on Facebook. Original article on Space.com.
Join our Space Forums to keep talking space on the latest missions, night sky and more! And if you have a news tip, correction or comment, let us know at: community@space.com.
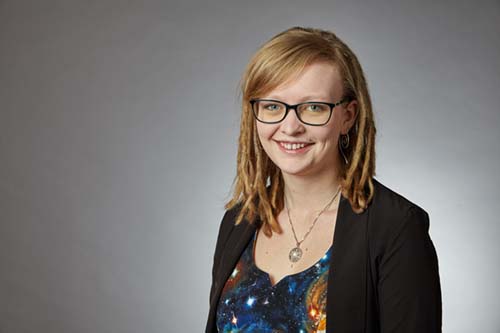
Hanneke Weitering is a multimedia journalist in the Pacific Northwest reporting on the future of aviation at FutureFlight.aero and Aviation International News and was previously the Editor for Spaceflight and Astronomy news here at Space.com. As an editor with over 10 years of experience in science journalism she has previously written for Scholastic Classroom Magazines, MedPage Today and The Joint Institute for Computational Sciences at Oak Ridge National Laboratory. After studying physics at the University of Tennessee in her hometown of Knoxville, she earned her graduate degree in Science, Health and Environmental Reporting (SHERP) from New York University. Hanneke joined the Space.com team in 2016 as a staff writer and producer, covering topics including spaceflight and astronomy. She currently lives in Seattle, home of the Space Needle, with her cat and two snakes. In her spare time, Hanneke enjoys exploring the Rocky Mountains, basking in nature and looking for dark skies to gaze at the cosmos.