For Close-Knit Planets, Sharing Life Could Be Easy
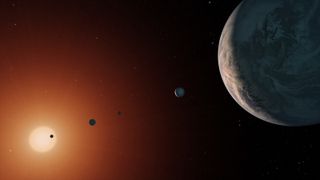
How could life be shared between planets in close proximity to one another? This question has received a greater insight thanks to new analytics based on previously known and new calculations.
The findings from this new research are helping scientists understand how likely life would be on a given planet in such tight-knit systems if that world shows signs of habitability.
This approach began with a blasphemous-at-the-time idea: that life exists throughout the universe and can travel without supernatural interference. Anaxagoras, a 5th-century B.C. Greek philosopher, called this concept "panspermia." Kelvin, Helmholtz and Arrhenius advanced the idea in the 19th and 20th centuries by examining how life could be carried to and from Earth. In 2009, Stephen Hawking went beyond our solar system with the idea when he suggested that "life could spread from planet to planet or from stellar system to stellar system, carried on meteors." [5 Bold Claims of Alien Life]
Dimitri Veras, an astrophysicist at the University of Warwick in England and lead author of a recent paper on the subject, said, "Within the last century, [panspermia] has been focused on life transport within the solar system, including Earth."
The TRAPPIST-1 solar system, which is 39 light-years from Earth and includes seven planets packed into an orbit smaller than Mercury's, changes this Earth-centric idea. This system's sun is an ultracool red dwarf. So, even though the seven nearby planets orbit closely, they are possibly all still in the habitable zone, to varying degrees depending upon the makeup of their atmospheres. That makes this system a perfect model for exploring the idea of panspermia, per Hawking, anywhere in the universe.
Three stages
But back to our solar system, where the "foundation for panspermia-related processes has been established," Veras' paper said. That includes evidence that life can survive the three stages of traveling from one planet to another: initial ejection, the journey through space between planets and impact onto a new planet. Each stage presents challenges to the survival of life.
Veras wanted to create an analytical system to quantify each of these parts to create a better understanding of the probability of the whole process occurring.
Get the Space.com Newsletter
Breaking space news, the latest updates on rocket launches, skywatching events and more!
He had some information to start with: Microbes can survive ejection from a planet with life on it, as per previous studies, and even a voyage through interplanetary space, if shielded from the radiation and cold. Less is known about how well a microbe that endured space travel could survive impact on a new planet, which would be necessary for life to complete the voyage from one planet to another.
Because impact includes more unknowns than ejection and transit between planets, Veras had less-detailed information to work with in this area of his calculations.
"The physics of re-entry features complexities that are not present with the ejection and voyage phases through space," he said. "For example, frictional heating during re-entry can lead to the formation of a fusion crust [the outer layer of the meteorite that melts and ablates during atmospheric entry] on the surface of the meteorite."
To figure out how to calculate the tricky physics of atmospheric entry onto a new planet, Veras turned to some already-available math. He told Astrobiology Magazine that "Equations regarding the physics of impact have already been established and used for solar system applications, [so] we converted those for use in a general extrasolar system."
To understand the probability of ejected material traveling from one planet to another, Veras combined his equations into analytics. This way, he could figure out the whole system of panspermia, not just parts of it.
"Usually, the dynamics of panspermia is studied with numerical simulations. However, these can be slow to run and must be tailored to an individual system," Veras said. "Alternatively, analytics are much faster to use and are general enough to be applicable to a wide variety of systems." [Exoplanet Discovery: The 7 Earth-Size Planets of TRAPPIST-1 in Pictures]
Sharing life
These days, scientists know of an observable multiplanet system, TRAPPIST-1, with more than one world in the habitable zone. So, astrobiologists can use Veras' analytics to understand the probability of life being shared between planets in these extrasolar locales.
The closeness of the planets in this new system means that the chance that they can share material is high. If life began on one of the planets, can Veras' analytics tell us whether that life may have traveled to another given planet, thanks to panspermia? His equations are not meant to do that, he said. Veras said that they are "not exact" but instead "provide a sufficiently good approximation." Rather, their aim is to give astrobiologists another tool with which to assess new planetary systems.
Amaya Moro-Martin, an astronomer at the Space Telescope Science Institute in Maryland who has published a paper on the probability of panspermia between different planetary systems, said Veras' analytics are "An impressive piece of work." This analytic approach "takes into account a wide range of physical processes that are involved in panspermia," Moro-Martin said.
Looking forward, Moro-Martin said Veras' work will be useful for when new exoplanet systems are discovered. "The framework that it establishes will help others assess whether, from the dynamical point of view, panspermia could have been feasible, given the system characteristics," said Moro-Martin, who was not involved in the Veras-led study.
Astrobiologists need to ensure that they are not limiting life to what's already known; aliens could look very different from what we expect, Moro-Martin said.
"The difficulty here is that the experiments that test survival against the hazards of outer space and atmospheric entry will be based on the organisms we are familiar with, and we have no clue what extrasolar organisms might be like," said Moro-Martin, "which opens a fascinating world of possibilities."
The study was published in September in the journal Astrobiology.
This story was provided by Astrobiology Magazine, a web-based publication sponsored by the NASA astrobiology program. This version of the story published on Space.com. Follow us @Spacedotcom or Facebook.
Join our Space Forums to keep talking space on the latest missions, night sky and more! And if you have a news tip, correction or comment, let us know at: community@space.com.