Weird quantum effect that can turn matter invisible finally demonstrated
The technique could be used to stop information loss from quantum computers.
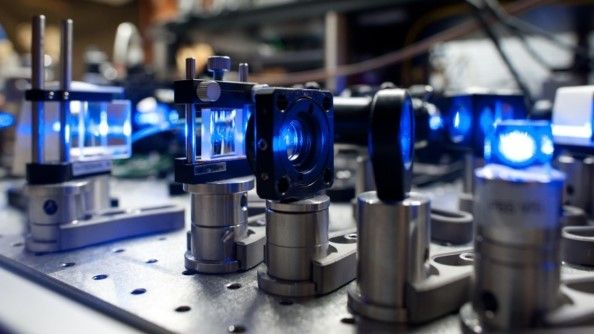
A weird quantum effect that was predicted decades ago has finally been demonstrated — if you make a cloud of gas cold and dense enough, you can make it invisible.
Scientists at the Massachusetts Institute of Technology (MIT) used lasers to squeeze and cool lithium gas to densities and temperatures low enough that it scattered less light. If they can cool the cloud even closer to absolute zero (minus 459.67 degrees Fahrenheit, or minus 273.15 degrees Celsius), they say it will become completely invisible.
The bizarre effect is the first ever specific example of a quantum mechanical process called Pauli blocking.
"What we've observed is one very special and simple form of Pauli blocking, which is that it prevents an atom from what all atoms would naturally do: scatter light," study senior author Wolfgang Ketterle, a professor of physics at MIT, said in a statement. "This is the first clear observation that this effect exists, and it shows a new phenomenon in physics."
The new technique could be used to develop light-suppressing materials to prevent information loss in quantum computers.
Related: Now you see it: 6 tales of invisibility in pop culture
Pauli blocking comes from the Pauli exclusion principle, first formulated by the famed Austrian physicist Wolfgang Pauli in 1925. Pauli posited that all so-called fermion particles — like protons, neutrons and electrons — with the same quantum state as each other cannot exist in the same space.
Get the Space.com Newsletter
Breaking space news, the latest updates on rocket launches, skywatching events and more!
Because at the spooky quantum level there only are a finite number of energy states, this forces electrons in atoms to stack themselves into shells of higher energy levels that orbit ever farther around atomic nuclei. It also keeps the electrons of separate atoms apart from each other because, according to a 1967 paper co-authored by the famed physicist Freeman Dyson, without the exclusion principle all atoms would collapse together while erupting in an enormous release of energy.
These outcomes not only produce the startling variation of the elements of the periodic table but also prevent our feet, when planted on the dirt, from falling through the ground, taking us tumbling into the Earth's center.
The exclusion principle applies to atoms in a gas too. Usually, atoms in a gas cloud have a lot of space to bounce around in, meaning that even though they may be fermions bound by the Pauli exclusion principle, there are enough unoccupied energy levels for them to jump into for the principle to not significantly impede their movement. Send a photon, or light particle, into a relatively warm gas cloud and any atom it bumps into will be able to interact with it, absorbing its incoming momentum, recoiling to a different energy level, and scattering the photon away.
But cool a gas down, and you have a different story. Now the atoms lose energy, filling all of the lowest available states and forming a type of matter called a Fermi sea. The particles are now hemmed in by each other, unable to move up to higher energy levels or drop down to lower ones.
At this point they’re stacked in shells like seated concertgoers in a sold out arena and have nowhere to go if hit, the researchers explained. They're so packed, that the particles are no longer able to interact with light. Light that is sent in is Pauli blocked, and will simply pass straight through.
"An atom can only scatter a photon if it can absorb the force of its kick, by moving to another chair," Ketterle said. "If all other chairs are occupied, it no longer has the ability to absorb the kick and scatter the photon. So, the atom becomes transparent."
Related: 18 times quantum particles blew our minds
But getting an atomic cloud to this state is very difficult. It not only needs incredibly low temperatures but also requires the atoms to be squeezed to record densities. It was a delicate task, so after nabbing their gas inside an atomic trap, the researchers blasted it with a laser.
In this case, the researchers tuned the photons in the laser beam so that they collided only with atoms moving in the opposite direction to them, making the atoms slow and, therefore, cool down. The researchers froze their lithium cloud to 20 microkelvins, which is just above absolute zero. Then, they used a second, tightly focused laser to squeeze the atoms to a record density of roughly 1 quadrillion (1 followed by 15 zeros) atoms per cubic centimeter.
Then, to see how cloaked their supercooled atoms had become, the physicists shined a third and final laser beam — carefully calibrated so as to not alter the gas's temperature or density — at their atoms, using a hypersensitive camera to count the number of scattered photons. As their theory predicted, their cooled and squeezed atoms scattered 38% less light than those at room temperature, making them significantly dimmer.
Two other independent teams have also cooled down two other gases, namely potassium and strontium, to show the effect too. In the strontium experiment, the researchers Pauli blocked excited atoms to keep them in an excited state for longer. All three papers demonstrating Pauli blocking were published Nov.18 in the journal Science.
Now that researchers have finally demonstrated the Pauli blocking effect, they could eventually use it to develop materials that suppress light. This would be especially useful for improving the efficiency of quantum computers, which are currently hindered by quantum decoherence — the loss of quantum information (carried by light) to a computer's surroundings.
"Whenever we control the quantum world, like in quantum computers, light scattering is a problem and means that information is leaking out of your quantum computer," Ketterle said. "This is one way to suppress light scattering, and we are contributing to the general theme of controlling the atomic world."
Originally published on Live Science.
Join our Space Forums to keep talking space on the latest missions, night sky and more! And if you have a news tip, correction or comment, let us know at: community@space.com.
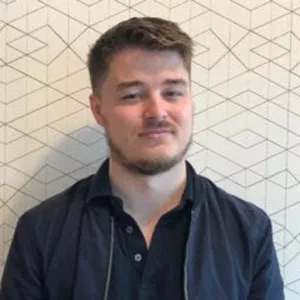
Ben Turner is a U.K. based staff writer at Live Science. He covers physics and astronomy, among other topics like weird animals and climate change. He graduated from University College London with a degree in particle physics before training as a journalist. When he's not writing, Ben enjoys reading literature, playing the guitar and embarrassing himself with chess.