Why NASA's Mars rover Perseverance will use nuclear power to keep itself warm
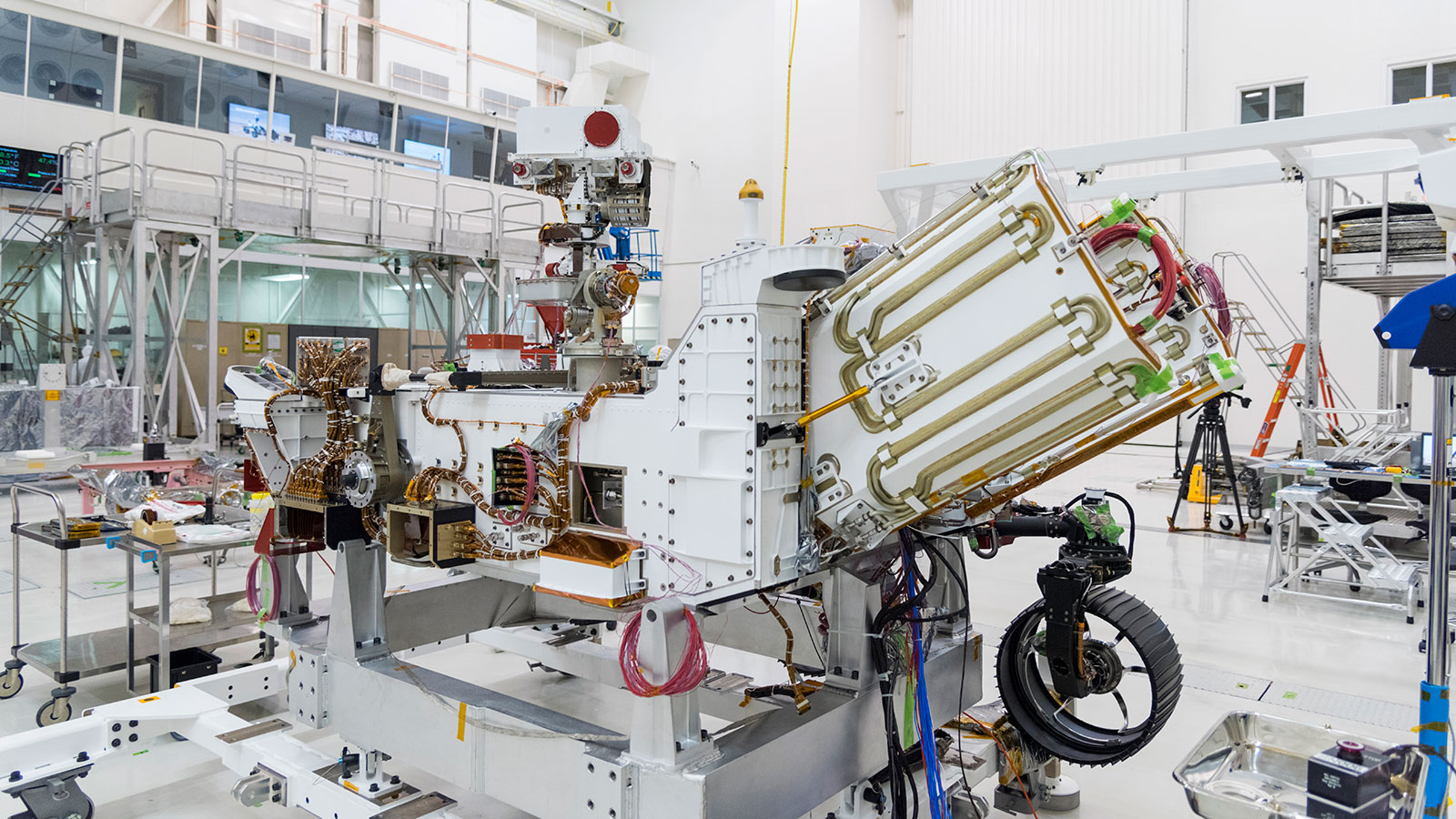
A spacecraft is only as strong as its power source, which is why when NASA was designing its Perseverance Mars rover, the agency turned to radioactive plutonium.
The plutonium that will be blasting off the planet on Thursday (July 30) isn't in the same form as is used for weapons, and it's well protected in case something happens to go wrong during the launch. But these plutonium units are a respected power source for spacecraft — NASA's Curiosity rover runs on a similar device.
"NASA likes to explore, and we have to explore in some very distant locations, dusty locations, dark locations and harsh environments," June Zakrajsek, a nuclear fuel expert at NASA's Glenn Research Center in Ohio, said in a Department of Energy (DOE) podcast about the Perseverance mission. "When we're in those kinds of environments, solar energy sometimes does not provide the power that we need. The light just does not get to those locations like we would need it."
Related: NASA's Mars 2020 Perseverance rover: Live updates
Some NASA missions to Mars have run on solar energy, of course — the InSight lander currently operating on the Red Planet bears solar panels, as did the twin Spirit and Opportunity rovers earlier this century. But Opportunity is a mascot for the weaknesses of solar energy at Mars, since the rover's end came when a massive global dust storm blocked it from tapping into the sun's light. Run a rover on nuclear power and you don't have to worry about that scenario.
So for the Perseverance rover, NASA turned to plutonium in a system called a Multi-Mission Radioisotope Thermoelectric Generator (MMRTG), which should be able to power the spacecraft for about 14 years.
"You don't have extension cords, you can't run out for a repairman," Bob Wham, a nuclear fuel expert at Oak Ridge National Laboratory, said in the same podcast. "You have to be totally reliable."
Get the Space.com Newsletter
Breaking space news, the latest updates on rocket launches, skywatching events and more!
Like the rest of the Perseverance rover, the MMRTG is based heavily on that of the Curiosity rover, which launched in 2011, landed on the Red Planet in 2012, and has been steadily chugging along ever since. Perseverance's MMRTG has been in the works for seven years, nearly as long as its predecessor has been powering Curiosity, and carries a price tag of $75 million, according to the DOE.
(Nuclear power sources of other varieties have also traveled to deep space on missions like the forty-year-old twin Voyager probes and the Cassini spacecraft that dived through Saturn's rings.)
Perseverance's MMRTG is designed to produce 110 watts of power, about the same as is used by a light bulb. The plutonium will decay, emitting heat that a generator converts into energy to power all of the rover's instruments, plus producing enough heat to protect the spacecraft from the freezing nights and winters on Mars.
The plutonium began as a different element entirely, neptunium, which scientists irradiated with neutrons in a nuclear reactor for nearly two months to convert it to the plutonium form needed for the MMRTG. The plutonium is then combined with ceramic, making a safer compound than is used in weapons.
Nevertheless, putting a nuclear power source at the tip of a rocket still prompts some cautionary measures. Most importantly, each pellet of plutonium is encased in iridium, which would contain the radioactive material if it fell back to Earth. According to NASA and the DOE, that's happened to space-bound nuclear power sources on three occasions, none of which caused any damage, with one of the power sources even being fished out of the ocean for later use on another mission.
In photos: NASA's Mars Perseverance rover mission to the Red Planet
NASA beefs up the mission control teams for such launches with additional personnel to coordinate any necessary response to the nuclear aspect of the mission. For the Perseverance launch, the government modeled a whole host of things that could go wrong on launch day — covering everything from an issue before liftoff that would have relatively compact geographic impact to a problem in Earth orbit that keeps the spacecraft from leaving for Mars.
Both of these scenarios have a probability below 0.1%, according to the government's models, and if an issue does occur during launch, those calculations suggest that even the most concentrated radiation exposure would be equivalent to about eight months of background radiation experienced by people living in the U.S.
And so Perseverance is sitting on the launchpad loaded with an MMRTG containing 32 hot, silvery lumps of fuel, ready to blast off to the Red Planet.
Unlike Curiosity's plutonium, some of that aboard Perseverance is relatively fresh and U.S.-made. The form of plutonium used on these missions began as a byproduct of nuclear weapon production processes, according to reporting by Slate after Curiosity's landing, and the U.S. government stopped creating its own supply of this plutonium in the 1980s, having decided it could access enough for its needs.
But lately, NASA has been stuck rationing out the power sources, which is why the DOE decided in 2015 to get back in the business of making plutonium — up to 14 ounces (400 grams) each year right now, with an eye on being able to make 3.3 lbs. (1.5 kilograms) each year by 2026, according to the DOE.
As to where that plutonium will go, one future nuclear-powered NASA mission is already in the works. The agency's Dragonfly mission, a drone bound for Saturn's strange large moon, Titan, will be powered by an MMRTG. That spacecraft is scheduled to launch in 2026.
Email Meghan Bartels at mbartels@space.com or follow her on Twitter @meghanbartels. Follow us on Twitter @Spacedotcom and on Facebook.
Join our Space Forums to keep talking space on the latest missions, night sky and more! And if you have a news tip, correction or comment, let us know at: community@space.com.
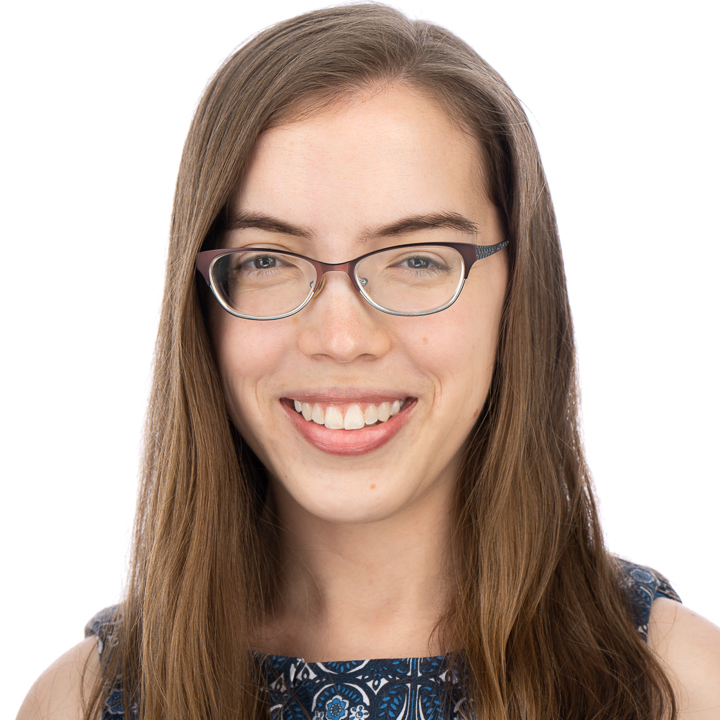
Meghan is a senior writer at Space.com and has more than five years' experience as a science journalist based in New York City. She joined Space.com in July 2018, with previous writing published in outlets including Newsweek and Audubon. Meghan earned an MA in science journalism from New York University and a BA in classics from Georgetown University, and in her free time she enjoys reading and visiting museums. Follow her on Twitter at @meghanbartels.
-
dfjchem721 At a price of $75 million for 110 watts, we won't be using these MMRTG*s for much else. No wonder space probes are so costly, even without this energy source. And powering the rover for 14 years is pretty amazing. Just hope the rest of the rover lasts that long.Reply
These particular RTGs actually use PU238, not the PU239 found in nuclear weapons, which is why they don't have a lot of this isotope, and which probably adds largely to their price tags.
Anybody with expertise in these, it would be nice to hear from you. There are different kinds of RTGs using various isotopes, both in use and under research. They are quite amazing devices!
* https://en.wikipedia.org/wiki/Radioisotope_thermoelectric_generator -
mfalkvidd Has anyone seen information on how the MMRTG is cooled during the 7 month journey to Mars? 2kW of heat is quite a lot.Reply -
Kdt Plutonium electric power isn't "nuclear." Nuclear power refers to modifying the nucleus of atoms either by splitting or combining. In typical nuclear power, the former is done by combining a large enough amount of radioactive material to form a "critical mass" which accelerates the natural decay process, causing massive amounts of heat, which then heats water into steam to turn a turbine (like most other power generation). That's not at all what a plutonium electric generator does. It just sits there and naturally decays, unaffected, which causes natural heat which is converted directly to electricity using a natural electric effect. It's not a "nuclear power source."Reply -
zloturtle
Space is like -400Fmfalkvidd said:Has anyone seen information on how the MMRTG is cooled during the 7 month journey to Mars? 2kW of heat is quite a lot. -
Unclear Engineer Kdt said:Plutonium electric power isn't "nuclear." Nuclear power refers to modifying the nucleus of atoms either by splitting or combining. In typical nuclear power, the former is done by combining a large enough amount of radioactive material to form a "critical mass" which accelerates the natural decay process, causing massive amounts of heat, which then heats water into steam to turn a turbine (like most other power generation). That's not at all what a plutonium electric generator does. It just sits there and naturally decays, unaffected, which causes natural heat which is converted directly to electricity using a natural electric effect. It's not a "nuclear power source."
I think this post is misleading, so I will try to provide a clearer explanation.
First, "nuclear" energy is energy that is contained in the nuclei of atoms, rather than in the electron clouds around the nuclei. What we refer to as chemical energy involves interaction of just the electron clouds, without changing the nuclei of the interacting atoms
Nuclear energy can be released by several mechanisms.
1. Natural radioactive decay. Elements are assigned to chemical names based on the number of protons in their nuclei, and then subdivided into isotopes of each chemical based on the number of neutrons in their nuclei. Some combinations of protons and neutrons are stable, and others are not, and tend to shoot out parts of the nucleus to get to a stable combination of protons and neutrons. This releases nuclear energy. Depending on the element and isotope, the particles shot out of the nucleus can be:
(A) alpha particles, which are a clump of 2 protons plus 2 neutrons, and those particle soon attract 2 electrons into orbits around them and become Helium-4 atoms, or
(B) beta particles that come from converting a neutron in the nucleus to a proton, and these beta particles/electrons just become normal electrons in orbits around atoms, and
(C) often gamma rays, which are very high energy light (photons), come out of the nucleus as the particles leave.
Capturing the fast moving particles and photons in the surrounding material heats the material and can be used to make useful work. The alpha particles are the easiest to capture, because their double positive charge makes them interact most strongly with surrounding matter, and that gives them a limited, very short range through that material. Beta particles have only a single charge, so they travel a bit further into surrounding material, but also have a range limit. Gamma rays have no charge, and interact only in a probabilistic way, so they penetrate in a manner such that their intensity is reduced by a fraction per unit distance, going a long way before their intensity is effectively reduced to near zero.
This distinction in how the different nuclear radiation emissions interact with matter makes it easy to provide shielding against alpha particles and beta particles, but not gamma rays. So, when engineers are designing a nuclear isotopic power source, they want something that provides a lot of energy in a form that is easy to keep where they want it and easy to prevent from going where they don't want it - and that is something that emits only alpha particles, with no gamma rays. That is what Plutonium 238 does - emits high energy alpha particles and no gamma rays. So, it is a very desirable material for making heat that can be turned into power for use in space probes that cannot rely on solar power (because, for instance, needing to work in dusty environments like Mars, or going to regions of the solar system too far from the sun, like the Voyager probes are still doing 45 years after launch).
B. Nuclear energy can also be released from some atomic nuclei by splitting the nucleus with a neutron from outside the nucleus. That is called fission, and is what is commonly used in commercial nuclear power reactors here on Earth (and elsewhere). A few isotopes of very heavy atomic nuclei are so unstable that they spontaneously emit neutrons, as well as alpha particles and gamma rays. And those neutrons can sometimes hit some of the other heavy nuclei and cause them to fission, releasing a lot more energy than happens when just an alpha particle is released. Fission releases two (or occasionally three) lighter isotopes, plus 2 or 3 neutrons, and a lot of gamma rays. Nuclear fission reactors are designed to manage a chain reaction so that only one of those released neutrons from each fission ends up, on average, causing another fission of another nucleus of the fissionable material, with the rest getting absorbed elsewhere - thus creating a steady release of power in a controlled manor. (Atomic bombs are designed to use as many of the released neutrons as possible to cause additional fissions, making the energy release increase exponentially until the fissionable material is exhausted or widely dispersed - an explosion.) Fission leaves a mess of radioactive lighter atoms, emitting beta particles and gamma rays, so it is hard to shield surrounding things from the damaging effects or radiation. Shielding materials are heavy, so launching reactors and their shielding into orbit or beyond is expensive. But, some reactors have been put into orbit (e.g., the SNAP series) and may be used on moon bases in the future.
C. To complete the discussion, nuclear energy can also be released by fusing 2 light weight nuclei, such as hydrogen nuclei, to make heavier nuclei such as helium. That is what the Sun does with its tremendous pressure and temperature. It uses 4 light hydrogen atoms (one electron orbiting one proton) and squeezes them together so that 2 of the electrons combine with 2 of the protons to produce 2 neutrons, and those 2 neutrons combine with the other 2 protons to make the nucleus of helium-4 (which is the same thing as an alpha particle BTW). That releases a lot of energy. We use almost the same process here on earth to make hydrogen bombs, and hope to someday be able to use the fusion process to make rate-controlled reactions for generating electricity. I said that we use almost the same process here on Earth because we "cheat" by using already made neutrons. So, what we can do requires a lot less pressure and temperature than what the Sun does when it is making the neutrons. What we do on earth is use the neutrons that are already in the rare, unusually heavy isotopes of hydrogen, called Deuterium if there is one neutron in the nucleus with the proton, and Tritium if there are 2 neutrons in the hydrogen nucleus.
As said at the beginning, all 3 of these processes release energy from the nuclei of the atoms involved, not just the energy involved in the cloud of atoms surrounding the nuclei, so these are all nuclear energy sources.