Heavy Elements Key for Planet Formation, Study Suggests
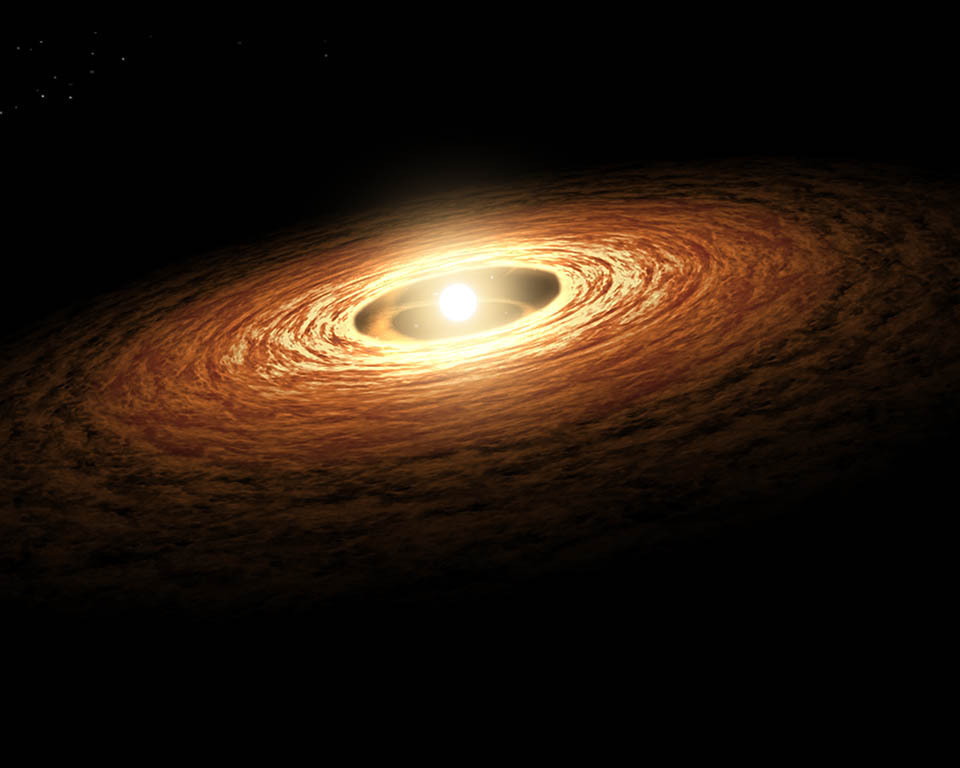
Planets form more commonly in star systems with relatively high concentrations of elements heavier than hydrogen and helium, a new study suggests.
Such heavier elements are necessary to form the dust grains and planetesimals that build planetary cores, according to the study, which was carried out by researchers Jarrett Johnson and Hui Li of Los Alamos National Laboratory in New Mexico.
Additionally, evidence suggests that the disks of dust that surround young stars don’t survive as long when the stars have lower concentrations of heavy elements, or lower "metallicities" in astronomers' jargon. The most likely reason for this shorter lifespan is that light from the star causes clouds of dust to evaporate.
The planet epoch
Our cosmic history has several defining epochs, one of which is the point at which star systems began to form planets. Heavy elements such as carbon, silicon and oxygen first needed to be created from huge star explosions called supernovas and the stellar cores of the first generations of stars before the first planets could form. [Supernova Photos: Great Images of Star Explosions]
"Our calculation is an estimate of the minimum amount of heavy elements that must be present in circumstellar disks before planets can form," Johnson said. "Because these heavy elements must be produced by the first stars in the universe, the first planets could only form around later generations of stars."
Understanding how the first planets formed provides crucial information about the early universe. Additionally, a better understanding of early planetary formation impacts many facets of astronomy, including the search for life elsewhere, researchers say.
Breaking space news, the latest updates on rocket launches, skywatching events and more!
According to Johnson and Li, a successful theory of planet formation should make predictions about the properties of the earliest planets and their host stars. Such a theory could be tested by studying very old planetary systems in our galaxy. The enrichment of gas with metals from supernovas is thought to affect not only planetary formation, but the formation of low-mass stars like our sun as well.
"A planet as massive and dense as the Earth could only form once stars and supernovae had enriched the gas with an abundance of heavy elements that is at least 10 percent that in the sun," Johnson said. "This suggests that many generations of stars had to form and evolve before habitable planets could form."
One important consideration for planetary formation is the dispersal rate of the circumstellar disk of gas and dust around a host star. Two of the more prominent mechanisms for dispersing a planetary disk are giant planet formation and photoevaporation by the host star.
Photoevaporation appears to be the more dominant process Observations show that low-metallicity disks have shorter lifetimes, which is bolstered by data showing higher-metallicity disks are better "shielded" from evaporation by a host star’s radiation.
Johnson and Li further assert that disks with higher metallicity tend to form a greater number of high-mass giant planets.
The lifetime of dust
In order to obtain estimates of the critical metallicity necessary for planet formation, Johnson and Li compared the lifetime of the disk and the length of time required for dust grains in the disk to settle. Basically, for a star system to form planets, the time required for dust grains to settle cannot exceed the disk's lifetime.
Since the settling time for dust grains depends on the density and temperature of the disk, which are related to the distance from the host star, the critical metallicity is also a function of distance from the host star.
"Our calculation is really fairly simple compared to many others, as we have focused only on what we believe are the key processes that set the timescale required for planetesimal formation at low metallicity," Johnson said. "These are the growth of dust grains into planetesimals and the destruction of the disk by the high-energy radiation from the host star. While the calculation is simple, it does show that current models of planet formation can in principle explain how the lowest-metallicity planets form."
The team notes several assumptions made in their comparisons with the data. The first assumption is that surface metallicity of the host star is the same as that of the protostellar disk from which it and its planets formed. Second, the team assumes circular planetary orbits. When orbits are highly eccentric, comparing the data to the theoretical predictions is more difficult. Lastly, the team assumes planets have not migrated inward toward their star from their initial place of birth in the disk.
Johnson and Li found that the formation of planetesimals can only take place once a minimum metallicity is reached in a protostellar disk. Since the earliest stars that formed in the universe (Population III stars) do not have the required metallicity to host planets, it is believed that the supernova explosions from such stars helped enrich subsequent (Population II) stars, some of which may still be in existence and could host planets.
The earliest planets
Based on their equations, the team finds that some of the earliest planets may have formed at a distance of 0.03 astronomical units from their parent star. One astronomical unit, or AU, is the distance from Earth to the sun, or about 93 million miles (150 million kilometers). For comparison, our solar system's innermost planet, Mercury, orbits at just under 0.4 AU.
Given the high temperatures likely at 0.03 AU (estimated at roughly 2,370 degrees Fahrenheit, or 1,300 degrees Celsius), the first planets probably were too hot to host life as we know it. [The Strangest Alien Planets]
"Interestingly, our results also suggest that the first Earth-like planets may have formed in the habitable zones of stars slightly more massive than the sun," Johnson said. "Because more massive stars burn out faster, it is possible that any life that evolved on these planets may have already perished with the death of its host star, which may have lived only 4 billion years compared to the 10 billion year lifetime expected for the sun."
Johnson and Li also note that the formation of Earth-like planets is not itself a sufficient prerequisite for life to take hold, stating that early galaxies contained numerous supernovas and black holes — both strong sources of radiation that would threaten life. Given the hostile conditions in the early universe, it is expected that conditions suitable for life were only present after early galaxy formation.
"However, with the wealth of new exoplanets being discovered and characterized, our theory of the minimum metallicity for planet formation may yet be challenged," Johnson said. "It will be exciting to see how [our model] holds up."
Johnson and Li’s research is scheduled to appear in the Astrophysical Journal.
This story was provided by Astrobiology Magazine, a web-based publication sponsored by the NASA astrobiology program.
Join our Space Forums to keep talking space on the latest missions, night sky and more! And if you have a news tip, correction or comment, let us know at: community@space.com.