
A tiny crystal device could boost gravitational wave detectors to reveal the birth cries of black holes
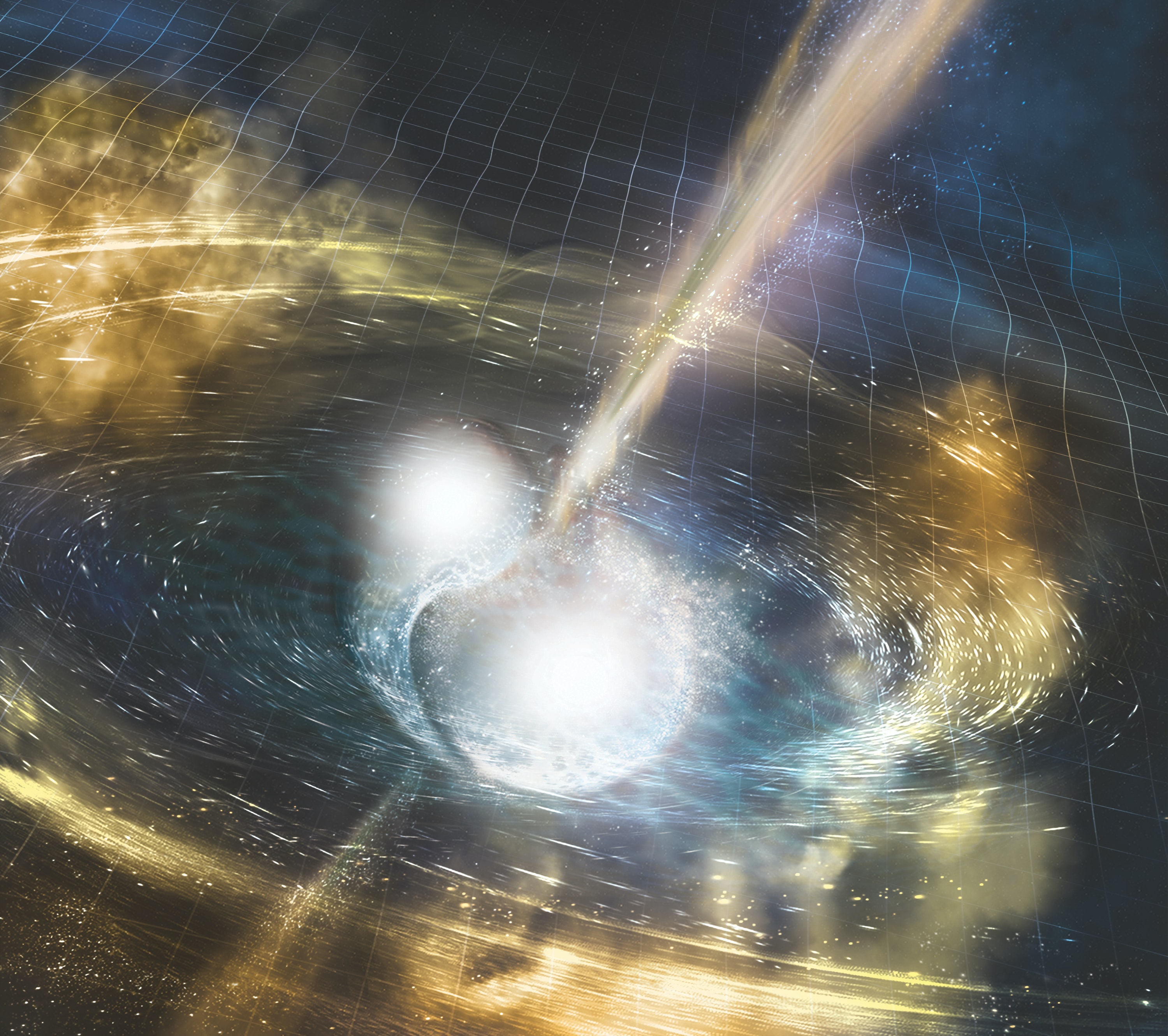
This article was originally published at The Conversation. The publication contributed the article to Space.com's Expert Voices: Op-Ed & Insights.
David Blair, Emeritus Professor, ARC Centre of Excellence for Gravitational Wave Discovery, OzGrav, University of Western Australia
In 2017, astronomers witnessed the birth of a black hole for the first time. Gravitational wave detectors picked up the ripples in spacetime caused by two neutron stars colliding to form the black hole, and other telescopes then observed the resulting explosion.
But the real nitty-gritty of how the black hole formed, the movements of matter in the instants before it was sealed away inside the black hole’s event horizon, went unobserved. That’s because the gravitational waves thrown off in these final moments had such a high frequency that our current detectors can’t pick them up.
Read more: At last, we've found gravitational waves from a collapsing pair of neutron stars
If you could observe ordinary matter as it turns into a black hole, you would be seeing something similar to the Big Bang played backwards. The scientists who design gravitational wave detectors have been hard at work to figure out how improve our detectors to make it possible.
Today our team is publishing a paper that shows how this can be done. Our proposal could make detectors 40 times more sensitive to the high frequencies we need, allowing astronomers to listen to matter as it forms a black hole.
Get the Space.com Newsletter
Breaking space news, the latest updates on rocket launches, skywatching events and more!
It involves creating weird new packets of energy (or “quanta”) that are a mix of two types of quantum vibrations. Devices based on this technology could be added to existing gravitational wave detectors to gain the extra sensitivity needed.
Quantum problems
Gravitational wave detectors such as the Laser Interferometer Gravitational-wave Observatory (LIGO) in the United States use lasers to measure incredibly small changes in the distance between two mirrors. Because they measure changes 1,000 times smaller than the size of a single proton, the effects of quantum mechanics – the physics of individual particles or quanta of energy – play an important role in the way these detectors work.
Two different kinds of quantum packets of energy are involved, both predicted by Albert Einstein. In 1905 he predicted that light comes in packets of energy that we call photons; two years later, he predicted that heat and sound energy come in packets of energy called phonons.
Photons are used widely in modern technology, but phonons are much trickier to harness. Individual phonons are usually swamped by vast numbers of random phonons that are the heat of their surroundings. In gravitational wave detectors, phonons bounce around inside the detector’s mirrors, degrading their sensitivity.
Read more: Australia's part in the global effort to discover gravitational waves
Five years ago physicists realised you could solve the problem of insufficient sensitivity at high frequency with devices that combine phonons with photons. They showed that devices in which energy is carried in quantum packets that share the properties of both phonons and photons can have quite remarkable properties.
These devices would involve a radical change to a familiar concept called “resonant amplification”. Resonant amplification is what you do when you push a playground swing: if you push at the right time, all your small pushes create big swinging.
The new device, called a “white light cavity”, would amplify all frequencies equally. This is like a swing that you could push any old time and still end up with big results.
However, nobody has yet worked out how to make one of these devices, because the phonons inside it would be overwhelmed by random vibrations caused by heat.
Quantum solutions
In our paper, published in Communications Physics, we show how two different projects currently under way could do the job.
The Niels Bohr Institute in Copenhagen has been developing devices called phononic crystals, in which thermal vibrations are controlled by a crystal-like structure cut into a thin membrane. The Australian Centre of Excellence for Engineered Quantum Systems has also demonstrated an alternative system in which phonons are trapped inside an ultrapure quartz lens.
We show both of these systems satisfy the requirements for creating the “negative dispersion” – which spreads light frequencies in a reverse rainbow pattern – needed for white light cavities.
Both systems, when added to the back end of existing gravitational wave detectors, would improve the sensitivity at frequencies of a few kilohertz by the 40 times or more needed for listening to the birth of a black hole.
What's next?
Our research does not represent an instant solution to improving gravitational wave detectors. There are enormous experimental challenges in making such devices into practical tools. But it does offer a route to the 40-fold improvement of gravitational wave detectors needed for observing black hole births.
Astrophysicists have predicted complex gravitational waveforms created by the convulsions of neutron stars as they form black holes. These gravitational waves could allow us to listen in to the nuclear physics of a collapsing neutron star.
For example, it has been shown that they can clearly reveal whether the neutrons in the star remain as neutrons or whether they break up into a sea of quarks, the tiniest subatomic particles of all. If we could observe neutrons turning into quarks and then disappearing into the black hole singularity, it would be the exact reverse of the Big Bang where out of the singularity, the particles emerged which went on to create our universe.
This article is republished from The Conversation under a Creative Commons license. Read the original article.
Follow all of the Expert Voices issues and debates — and become part of the discussion — on Facebook and Twitter. The views expressed are those of the author and do not necessarily reflect the views of the publisher.
Join our Space Forums to keep talking space on the latest missions, night sky and more! And if you have a news tip, correction or comment, let us know at: community@space.com.
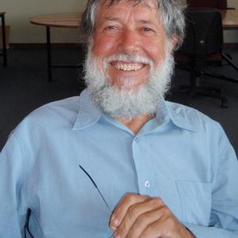
David Blair is an emeritus professor of physics at the University of Western Australia and member of Australian Research Council Centre of Excellence for Gravitational Wave Discovery (OzGrav). He leads an international project aiming to introduce Einsteinian concepts into school education at an early age.
His work has focused on the methods for the detection of gravitational waves. He built the first gravitational wave detector in Australia, and invented the sapphire clock, which was commercialised for precision radar systems as well as being used in metrology labs around the world.
He is a winner of the ANZAAS Medal, the Walter Boas Medal, the Breakthrough Prize, the Clunies Ross Medal for Science and Technology, and the Eureka Prize for public understanding of science.He was West Australian Scientist of the Year in 2007.