Distant 'teenage galaxies' surprise astronomers with unexpected heavy elements
These galaxies appear to be far hotter than expected as well.
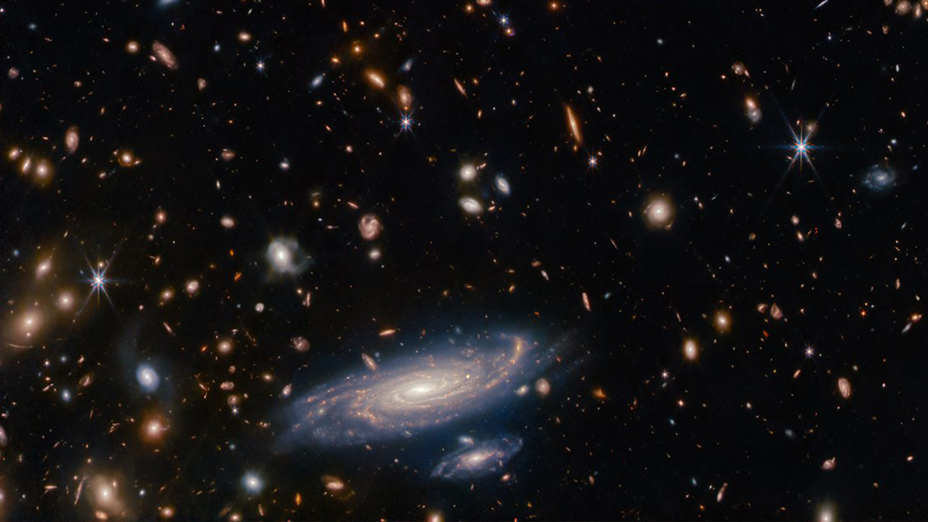
The deeper we look into space, the further back in time we see. Light emanating from some of the younger galaxies in our universe has to travel for billions of years to reach us, getting picked up by our instruments, rich with information from the cosmic dawn. And not only can this light tell us where we have come from, but where we might be headed.
To understand the evolution of several of these early universe, "teenage" galaxies, a Northwestern University-led team of astrophysicists have inspected data from the James Webb Space Telescope (JWST), which gazed back to realms that formed just two-to-three billions years after the Big Bang.
The observations have thrown up some intriguing surprises.
Specifically, the team analyzed results from the Chemical Evolution Constrained using Ionized Lines in Interstellar Aurorae (CECILIA) Survey to find that, not only do these galaxies appear hotter than expected, but they also seem to host heavy elements, like nickel.
Related: The James Webb Space Telescope catches distant young galaxy devouring its neighbors
The researchers focused on 33 distant galaxies for a continuous 30 hour period. They then combined wavelengths of light collected from 23 of those galaxies to create a composite picture of what's happening in these structures — these spectra contain clues regarding things like their average temperatures and what elements might be lurking within.
"This washes out the details of individual galaxies but gives us a better sense of an average galaxy. It also allows us to see fainter features," Allison Strom, lead author of the study and assistant professor of physics and astronomy at Northwestern University, said in a statement.
Get the Space.com Newsletter
Breaking space news, the latest updates on rocket launches, skywatching events and more!
The composite picture of the galaxies contained eight identifiable elements: Hydrogen, helium, nitrogen, oxygen, silicon, sulfur, argon and nickel. While the lighter elements were expected, the presence of nickel, which is heavier than iron in the periodic table, came as somewhat of a surprise.
"Never in my wildest dreams did I imagine we would see nickel," Strom said.
Even in older, nearby galaxies, nickel rarely is observed — and that's after multiple life cycles of stars, meaning multiple rounds of supernovas, and the opportunity for heavier elements to synthesize and spread throughout the galaxy.
"No one ever talks about observing nickel. Elements have to be glowing in gas in order for us to see them. So, in order for us to see nickel, there may be something unique about the stars within the galaxies," Strom said.
Strom believes the higher observed temperatures in these early galaxies might be connected to their curious chemical composition somehow: "Ultimately, the fact that we see a higher characteristic temperature is just another manifestation of their different chemical DNA because the temperature and chemistry of gas in galaxies are intrinsically linked."
The study was published Nov. 20 in the journal The Astrophysical Journal Letters.
Join our Space Forums to keep talking space on the latest missions, night sky and more! And if you have a news tip, correction or comment, let us know at: community@space.com.
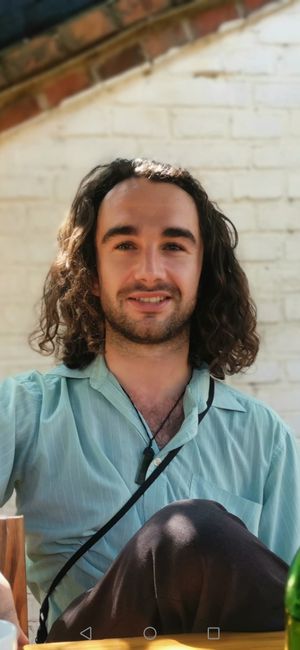
Conor Feehly is a New Zealand-based science writer. He has earned a master's in science communication from the University of Otago, Dunedin. His writing has appeared in Cosmos Magazine, Discover Magazine and ScienceAlert. His writing largely covers topics relating to neuroscience and psychology, although he also enjoys writing about a number of scientific subjects ranging from astrophysics to archaeology.
-
rod Intriguing report and JWST observations. The paper cited is, CECILIA: The Faint Emission Line Spectrum of z ∼ 2–3 Star-forming Galaxies, https://iopscience.iop.org/article/10.3847/2041-8213/ad07dcReply
The cosmology calculators can show the age of the Universe at z and the look back time distance, as well as comoving radial distances.
https://lambda.gsfc.nasa.gov/toolbox/calculators.html
z of 3 shows, "The age at redshift z was 2.172 Gyr." "The light travel time was 11.550 Gyr."
Apparently, JWST does not observe metal free gas in large redshift objects or Population III stars confirmed. The BB model requires pristine, primordial gas clouds created that later evolve into the Universe we see today including planets like Earth, teeming with life. -
Helio These, perhaps, are the farthest seen emissions of heavier elements, but I don't consider these emissions to be from large redshifts.Reply
Their conclusion states:
"We have reported the first results from the CECILIA program (JWST PID 2593), which obtained ultra-deep ∼30 hr NIRSpec/G235M observations of 33 star-forming galaxies at z ∼ 1–3.
...
We significantly detect emission lines of eight different elements (H, He, N, O, Si, S, Ar, and Ni)..."
With the JWST, we are seeing objects as far out as z = 13, IIRC.
As Rob notes, even at z = 3, it was 2,172 million years of age. Given enough telescopic power, if stars formed at the earliest moments some models predict, and if they go SN, with their predicted very short life-span then we should be able, in principle, to see spectra from much greater redshifts...someday. Or am I missing something? -
billslugg We have a gap here. At the frequency of CMBR, we have amplifiers that can detect and amplify the EM. At infrared wavelengths we use detectors that create a current when electrons are knocked free. The problem is there is a bandwidth in between the shortest radio waves we can amplify with the tiniest transistors, and the longest wavelength we can detect with semiconductors. This inaccessible zone has the information you are asking for.Reply
Highest frequency EM can be amplified by a transistor is 1 THz which is 3 cm.
The longest wavelength infrared detector on JWST is 28.5 microns, which is 10 THz.
We have no way to observe the band from 1 THz to 10 THz in frequency.
This band is from 28.5 microns up to 30,000 microns in wavelength.
This gap includes the time period from 780,000 years to 400,000,000 years. -
Classical Motion I always thought light went from ~400 THz to 800 THz. IR ~ 300-400 THz. I have heard some say IR goes from very low THz up to light. I call 1 to 300 THz the THz band. With some far IR at the top of the band. The 1 to 300 THz band has always interested me, the unknown and unexplored region. I would have thought these frequencies were local nucleus bonding frequencies, and not many emissions should be found in space.Reply
Very surprised to hear that 1-10 THz is the only missing part. Does optics bring us down to 10 THz? I must of missed something.
What does the 10 to 300 THz band look like from space? If the space expansion theory is true, wouldn't that band be full of down shifted light spectrums from past distances?
If I follow this right, the BB was the first(oldest) and the largest expansion. Giving that first light has a down shift to less than 1 THz. CBR. (a ridiculous statement)This should be the largest shift, meaning the lowest shift. And as we look at farther out stars, those star spectrums should shift down with distance. That means that the 10-300 THz band, should have repeated downshifts within it. From the different distances. Should be a repeatable pattern. Easy to see. The band should be busy.
Why do stars give us a spectrum, a slice, and not the whole EM spectrum? I believe it is due to superposition. And superposition takes many emitters. Such as zillions of dipoles in liquid plasma. Structure size determines emission frequency. We need smaller emitters for xray and gamma. And we need larger structures for below IR frequencies. So to get the same intensity, so that we can get the same range, many single electrons need to emit at same time for xray and many protons for gamma. These are rare compared to light. And the amount of larger structures, timed for the lower frequencies.......should even be rarer.
And I have another question about this down shift. Light has a band spread of 400 THZ.
Please tell me........where is that 400 THz bandwidth.....going to down shift too? Where is the room to downshift it into? Light is wide, it's fat. There is not enough frequency to shift down in to.
Does it shrink when it shifts? What was the bandwidth of the BB? Did that light shrink down to a skinny GHz signal?
Something is not right. -
rod In post #4, billslugg said, "This gap includes the time period from 780,000 years to 400,000,000 years."Reply
Cosmology calculators show the redshift for a universe 1 Myr or about is some 600. "The age at redshift z was 1.009 Myr." "The light travel time was 13.721 Gyr."
https://lambda.gsfc.nasa.gov/toolbox/calculators.html
I have not seen any reports confirming objects with such large redshifts or metal free gas seen in nature that the BB model requires. -
billslugg "Very surprised to hear that 1-10 THz is the only missing part. Does optics bring us down to 10 THz? I must of missed something." - Classical MotionReply
Anything below 1 THz is accessible by radio receivers.
Anything above 10 THz can be seen by IR detectors, human eye, X-ray detectors, gamma ray detectors.
The range from 1 to 10 THz is not accessible to our sensors. We cannot see what happened after 780,000 years and before 400 million years because the visible light emitted at that time has stretched out to be in the inaccessible zone.
If you could invent a sensor in the 1 to 10 THz range you could probably get a Nobel Prize. -
billslugg A z of ten is 449 million years ago. The EM wave here at Earth would be ~25 microns. We can image that using far infrared detectors.Reply -
Helio
Yep, I realized my confusion as soon as I got in my car. I somehow associated their z < 3 with a sensor limit. I now have had coffee, so I'm good to go. ;)billslugg said:A z of ten is 449 million years ago. The EM wave here at Earth would be ~25 microns. We can image that using far infrared detectors. -
Roger M. Pearlman Aligns best within Pearlman YeC SPIRAL and HTP hypotheses. Hyper-dense proto-galactic formation PRIOR to the hyper cosmic expansion epoch. We see the light that departed these and (all else at and beyond SPIRAL LY radius I) by the end of 4/365.25 (SPIRAL LY radius i) a fraction into history. During day 4. i = 6k rounded light years so same number of years ago. Reference Pearlman YeC SPIRAL cosmological redshift hypothesis and model at ResearchGate. Follow, enjoy, test, recommend and share to advance scienceReply