How did Earth form?
Earth's origins remain a conundrum.
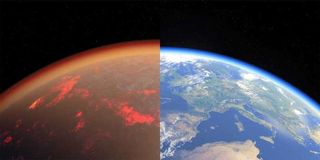
Earth's formation remains a strange, scientific mystery.
We live on a planet in a solar system with seven other planets and have discovered thousands of exoplanets to date. But how planets like Earth form still remains a subject of great debate.
Currently, there are two leading theories on planetary formation. Scientists continue to study planets in and out of our solar system in an effort to better understand which of these theories most accurately describes how the solar system and its planets formed.
The first and most widely accepted theory is the core accretion model, which works well to explain the formation of terrestrial planets like Earth but doesn't fully account for giant planets. The second theory, called the disk instability method, may account for the creation of larger planets. These two leading theories are joined by the pebble accretion theory which helps to additionally explain how different objects might form.
Related: Earth's layers: Exploring our planet inside and out
What is the core accretion model?
Approximately 4.6 billion years ago, our solar system was just a cloud of dust and gas known as a solar nebula. Gravity collapsed the material in on itself as it began to spin, condensing the matter and forming the sun in the center of the nebula.
With the sun beginning to form, the remaining material started to clump up. Small particles drew together, bound by the force of gravity, into larger particles. The solar wind, a constant stream of charged particles that emanate from the sun's upper atmosphere, swept away lighter elements, such as hydrogen and helium.
This left behind heavy, rocky materials that formed smaller terrestrial worlds like Earth. And farther away from the sun, the solar wind had less of an impact on lighter elements which allowed these elements to coalesce into gas giants. This process created our solar system's asteroids, comets, planets and moons.
Earth's rocky core formed first, with heavy elements colliding and binding together. Dense material sank to the protoplanet's center while lighter material built up the crust. Earth's magnetic field is thought to have likely formed around this time.
Early in its evolution, Earth suffered an impact by a large body that catapulted pieces of the young planet's mantle into space. Gravity pulled many of these pieces together to form the moon, which took up orbit around its creator.
The flow of the mantle beneath Earth's crust causes plate tectonics, the movement of the large plates of rock on the planet's surface. Collisions and friction gave rise to mountains and volcanoes, which began to spew gases.
When Earth first formed it had barely any atmosphere. Its atmosphere began to form as the planet started to cool and gravity captured gases from Earth's volcanoes.
While the population of comets and asteroids passing through the inner solar system is sparse today, they were more abundant when the planets and sun were young. Collisions between these cosmic bodies likely deposited much of the water on Earth's surface.
Our planet lies in what is known as the Goldilocks zone, a region surrounding a star that is close enough for liquid water to exist on a planet's surface, with water neither freezing nor evaporating. Many scientists think that being in this zone, and the presence of liquid water, plays a key role in the existence of life.
In observing exoplanets, scientists think that this core accretion model fits as the dominant formation process.
Stars with more "metals" — a term astronomers use for all chemical elements heavier than hydrogen and helium — in their cores host more giant planets than their metal-poor cousins. According to NASA, core accretion suggests that small, rocky worlds should be more common than the more massive gas giants.
One finding that has helped to strengthen core accretion's legitimacy as an explanation for planet formation is the 2005 discovery of a giant planet with a massive core orbiting the sun-like star HD 149026.
"This is a confirmation of the core accretion theory for planet formation and evidence that planets of this kind should exist in abundance," said Greg Henry in a press release. Henry, an astronomer at Tennessee State University, Nashville, detected the dimming of the star.
In 2019, the European Space Agency launched the CHaracterising ExOPlanet Satellite (CHEOPS), which was designed to study exoplanets ranging in sizes from super-Earths to Neptune. With missions like this and others, scientists aim to study distant worlds to grow their understanding of how planets in different solar systems likely formed.
"In the core accretion scenario, the core of a planet must reach a critical mass before it is able to accrete gas in a runaway fashion," said the CHEOPS team. "This critical mass depends upon many physical variables, among the most important of which is the rate of planetesimals accretion."
Related: How fast is Earth moving?
What is the disk instability model?
While the core accretion model works for terrestrial planets, gas giants would need to evolve rapidly to grab hold of the significant mass of lighter gases they contain. But simulations with that model have not been able to account for this rapid formation. In those simulations, the process takes several million years, which is longer than light gases were available in the early solar system.
But the core accretion model isn't the only explanation for how planets might come to be.
According to a newer theory, disk instability, clumps of dust and gas bind together early in the solar system's existence. Over time, these clumps can slowly compact into a giant planet. These planets can form faster than those that form within the core accretion explanation, sometimes in as little as a thousand years, which allows them to trap the rapidly-vanishing lighter gases. These planets also quickly reach an orbit-stabilizing mass that keeps them from death-marching into the sun.
According to exoplanetary astronomer Paul Wilson, if disk instability dominates the formation of planets, it should produce a wide number of worlds at large orders. The four giant planets orbiting at significant distances around the star HD 9799 provides observational evidence for disk instability.
Fomalhaut b, an exoplanet with a 2,000-year orbit around its star, could serve as an example of a world formed through disk instability, though the planet could also have been ejected due to interactions with its neighbors.
What is pebble accretion?
The disk instability model contends with the core accretion model's issue with time; specifically how quickly massive gas giants would have to grab lighter components. But another, recent model known as pebble accretion, also helps to fill in this explanatory gap.
In this model, researchers have shown how smaller, pebble-sized objects could have fused together to build giant planets up to 1000 times faster than in other explanations.
"This is the first model that we know about that you start out with a pretty simple structure for the solar nebula from which planets form, and end up with the giant-planet system that we see," Harold Levison, an astronomer at the Southwest Research Institute (SwRI) in Colorado and lead study author of a paper describing and exploring the model, told Space.com in 2015.
A few years earlier, in 2012, researchers Michiel Lambrechts and Anders Johansen from Lund University in Sweden proposed that tiny pebbles, once written off, held the key to rapidly building giant planets.
"They showed that the leftover pebbles from this formation process, which previously were thought to be unimportant, could actually be a huge solution to the planet-forming problem," Levison said.
Levison and his team built on that research to model more precisely how tiny pebbles could form planets seen in the galaxy today. In previous simulations, both large and medium-sized objects consumed their pebble-sized cousins at a relatively constant rate, but Levison's simulations suggest that the larger objects acted more like bullies, snatching away pebbles from the mid-sized masses to grow at a much faster rate.
"The larger objects now tend to scatter the smaller ones more than the smaller ones scatter them back, so the smaller ones end up getting scattered out of the pebble disk," study co-author Katherine Kretke, also from SwRI, told Space.com. "The bigger guy basically bullies the smaller one so they can eat all the pebbles themselves, and they can continue to grow up to form the cores of the giant planets."
As scientists continue to study planets inside and outside of the solar system, they will better understand how Earth and its siblings formed.
Additional resources
- Visit NASA's hub for understanding Earth as a planet.
- Explore NASA's kid-friendly resources for learning about Earth.
- Browse NASA's hub for understanding exoplanets.
Follow us at @Spacedotcom, Facebook or Google+.
This article was updated on Jan. 5, 2022 by Space.com senior writer Chelsea Gohd
Join our Space Forums to keep talking space on the latest missions, night sky and more! And if you have a news tip, correction or comment, let us know at: community@space.com.
Get the Space.com Newsletter
Breaking space news, the latest updates on rocket launches, skywatching events and more!
Nola Taylor Tillman is a contributing writer for Space.com. She loves all things space and astronomy-related, and enjoys the opportunity to learn more. She has a Bachelor’s degree in English and Astrophysics from Agnes Scott college and served as an intern at Sky & Telescope magazine. In her free time, she homeschools her four children. Follow her on Twitter at @NolaTRedd