
Detecting Ripples in Space-Time, with a Little Help from Einstein
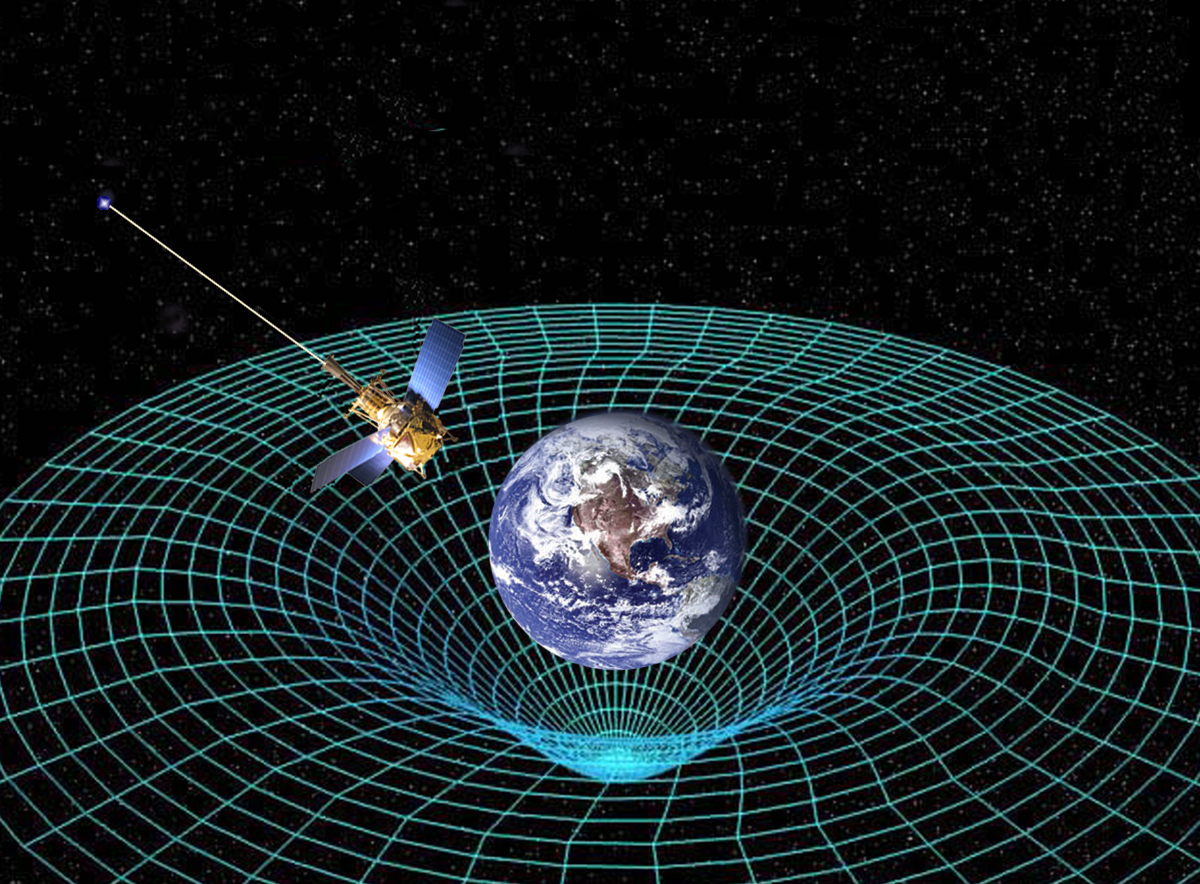
Marco Cavaglia is an associate professor of Physics and Astronomy at the University of Mississippi and a member of the LIGO team. Kai Staats is a filmmaker and an applied mathematics masters student at the University of Cape Town. Shivaraj Kandhasamy is a postdoctoral researcher collaborating with Cavaglia in his laboratory. The authors contributed this article to Space.com's Expert Voices: Op-Ed & Insights.
We look out into the cosmos with optical and radio telescopes and see distant star systems and far more distant galaxies. We can discern, to various degrees, how those systems were formed, and how they evolved over time. High-energy astrophysics observatories shed another kind of light onto those subjects, providing further insight to their associated, physical phenomena.
But to date, science has yet to explore the inner structure of a neutron star, nor has anyone witnessed the final moments as two black holes collide. With gravitational waves, we have a unique opportunity to see the universe in a whole new way. [LIGO Generations Trailer]
The Laser Interferometer Gravitational-Wave Observatory (LIGO) was designed to provide that new view. With detectors in Washington State and Louisiana, LIGO has recently completed a major upgrade. In the coming years, the Advanced LIGO instruments are expected to make gravitational wave detections a routine occurrence.
Of gravity and gravitational waves
In 1915, Albert Einstein published what is now the universally accepted theory of gravity: the general theory of relativity. An extension of the special theory of relativity, general relativity predicts that massive objects curve space-time around them.
A commonly used analogy is a ball sitting on a stretched rubber membrane. The ball produces a lower point (a depression) on the membrane such that a small bead rolling close to the ball no longer moves in a straight line, but along on a curved path — the bead is "caught in the gravitational well" of the ball.
"One can relate this analogy to our solar system, with the ball as the sun and the bead as a planet," said Hunter Gabbard, research assistant at the University of Mississippi. "Since Newton's first law of motion associates change of speed and direction to a force, the planet's response to the space-time curvature produced by the sun may be interpreted by an observer as a force: gravity. If the ball were removed from the membrane, the bead would roll on a straight line with constant speed, neglecting friction, of course. Similarly, if the sun suddenly disappeared, the planets in the solar system would be free from the gravitational well of the sun and move in a straight line with constant speed, off into interstellar space."
Get the Space.com Newsletter
Breaking space news, the latest updates on rocket launches, skywatching events and more!
Both Newton's and Einstein's theories explain how the planets revolve around the sun and tell us that the planets would fly off in straight lines if the sun were to vanish. However, the Newtonian theory describes space as immutable and all forces, including gravity, acting instantaneously. General relativity describes space and time as two dynamic aspects of the same entity, with changes in space-time traveling at the speed of light. Einstein's explanation of gravity has interesting consequences. For example, if the sun were to vanish now, planets would not fly off immediately.
Because of the finiteness of the speed at which gravity travels, it would take a finite period of time for the planets to feel the gravitational effect of the sun's disappearance. The Earth would continue in its current orbit for approximately 8 minutes — the time it takes for gravity to travel from the center of the solar system to Earth's orbit. Another consequence of general relativity, and perhaps the most amazing prediction of the theory, is the existence of gravitational waves.
According to Einstein's theory, any accelerated object produces dynamic, propagating perturbations of space-time. Just as you can create waves on the surface of a cup of coffee by stirring it with a spoon, the equations of general relativity say that matter can stir space-time if it is accelerated.
The concept of acceleration linked to wave generation is not a novelty of Einstein's theory. Half a century before Einstein, physicist James Clerk Maxwell had explained how electromagnetic waves could be generated through the acceleration of charges. What is different in general relativity is that gravitational waves are propagating distortions "of" space-time, rather than waves "in" space.
As with all waves in nature, from the sound waves of a trombone to the electromagnetic waves from your favorite radio station, gravitational waves carry energy. However, they interact with other forms of energy very weakly, so they don't lose a great deal of energy during their journey from distant cosmic sources. Once they are produced, they travel through the universe forever (well, almost . . .) carrying precious information about their origins — information that is unattainable by other means due to the incredibly weak nature of the signal. LIGO is the first instrument designed for this kind of detection.
How to catch a gravitational wave
So, how are gravitational waves detected?
Observation of the orbital change of the Hulse-Taylor binary star system, the first binary pulsar system ever discovered, has produced indirect evidence for gravitational waves. Similar to a satellite in orbit around our planet that sinks toward the Earth due to the loss of energy via atmospheric friction, the Hulse-Taylor pulsars (neutron stars) sink together. As the two stars in the Hulse-Taylor system get closer, they shed energy by stirring the space-time around them. This energy is lost in the form of gravitational waves.
By monitoring the motion of this binary pulsar beacon, scientists are able to test whether the rate of energy loss matches what one would expect to occur due to the emission of gravitational waves. The rate predicted by gravitational wave emission and the rate measured by monitoring their pulsating signals agree to extraordinary precision over the course of 30 years of observation. In 1993, Joseph Hooton Taylor, Jr. and Russell Hulse were awarded the Nobel prize in physics for their work in 1974 at the Arecibo radio telescope observatory.
The Hulse-Taylor pulsar system provides indisputable evidence of the existence of gravitational waves. Yet, this is not direct evidence — no one has observed an actual gravitational wave.
Following more than a decade of design, development and planning, with major funding support from the U.S. National Science Foundation, construction of the Laser Interferometer Gravitational-Wave Observatory (LIGO) began in 1999. The function of LIGO is to directly detect gravitational waves originating in distant galaxies.
LIGO facilities comprise two "L"-shaped structures, separated by hundreds of miles, each possessing two 2.5-mile-long (4 kilometers) arms. One of the facilities is in Hanford, Washington, and the other is in Livingston Parish, Louisiana. The "L" shape is dictated by each facility's detectors, laser interferometers.
The degree of precision required to "catch a gravitational wave" is indeed hard to imagine. Einstein did not believe such measurements could ever be made. To detect a typical cosmic gravitational wave on Earth, one must measure a change in distance of less than 10-19 meters (roughly 1/10,000th the diameter of a proton)!
Three LIGO detectors (two in Hanford and one in Livingston) came online in 2002. Following years of painstaking tuning and improvement, they operating at their design sensitivity from November 2005 to September 2007. Additional data run with enhanced versions of two of those detectors was completed in 2010. Dubbed Enhanced LIGO, the upgrade provided increased laser power, frequency-modulated radiation detection, output mode cleaner and in-vacuum readout hardware.
The primary purpose of the initial LIGO project was to demonstrate that researchers could build and operate instruments sensitive enough to detect gravitational waves. Before LIGO, no attempt had ever been made to detect gravitational waves using large-scale interferometers. The required technology did not exist when LIGO's construction began. The LIGO project was tantamount to building a completely new kind of scientific instrument — not exactly something that you can order on Amazon! While no detection was made by the initial LIGO, the first-generation LIGO instrument proved that the goal of directly detecting gravitational waves was achievable. And even without a detection, LIGO's data led to many astrophysical results.
One such example was related to a short, intense outburst of energetic gamma rays detected in the direction of the Andromeda galaxy by the Konus-Wind, Integral, Messenger, and Swift gamma-ray satellites on February 1, 2007. At the time of the observation of this gamma ray burst, called GRB070201, the LIGO instruments at the Hanford facility were collecting science data. Such a monumental, cosmic event occurring in a nearby galaxy should have generated gravitational waves easily measured by the LIGO. The non-observation of a gravitational-wave signal in the aftermath of the burst meant that GRB070201 did not originate in Andromeda. Other causes for the event, such as a soft gamma-ray repeater or a binary merger from a much further distance, were the most likely cause for the event.
In 2010, the engineers and technicians at both the Hanford and Livingston facilities engaged in the task of fully disassembling the Enhanced LIGO instruments in order to upgrade to a far more powerful configuration: Advanced LIGO.
That journey concluded a few weeks ago, on May 19, when LIGO's two advanced detectors were dedicated at a ceremony in Hanford. Advanced LIGO will begin collecting astrophysical data later this year.
Eventually, the machines will become 10 times more sensitive than their first-generation predecessors, reaching far beyond our local Virgo supercluster of galaxies and opening up a thousand-fold increase in the volume of space that LIGO can survey.
How an interferometer works
Interferometers are optical instruments that use light's interference property — specifically, to measure small differences in distance between two light paths. Due to their high accuracy, they are used widely in science and other industries to make very accurate measurements of both incredibly small and tremendously large distances.
The basic mechanism of a gravitational-wave interferometer is simple: A single laser beam is split into two beams traveling on different paths, which are then reflected by mirrors and then recombined into a single beam that strikes a detector.
The beams are carefully aligned out of phase to cancel each other out when they recombine. The two beams "destructively interfere" — hence the term "interferometer." If a gravitational wave warps space-time and changes the length of the beam paths, the two beams will no longer be fully out of phase. Even a minuscule change in space-time will place the light waves out of phase, producing a tiny amount of light that strikes the interferometer's detectors.
Of course, in real life, things are not so simple.
Because the gravitational-wave signals are identified by light-intensity variations at the output of the detector, having an ultrastable laser is very important. A system of input optics (IO) provides an initial stage of stabilization as the laser enters the interferometer. Within the input optics, the input mode cleaner (IMC) reduces fluctuations in the light entering the interferometer and provides frequency stabilization by filtering out unwanted laser modes.
The beam is then sent to the beam splitter (BS) that separates the beam into two beams, each of which is sent down a separate, perpendicular arm of the L-shaped detector. The beams reflect off end mirrors (ETMs), return to the beam splitter where they are redirected for the last time, and finally combine at the output photo-detector (labeled "GW readout"). Two input mirrors (ITMs) keep the laser light trapped in the arms for some time, effectively increasing the arm lengths, and thus the detector sensitivity.
If the laser spends twice as much time in each arm, the arm appears (to the laser) to be twice as long. Therefore, the sensitivity is doubled. If the laser traverses the total length 100 times, for approximately 1 millisecond, it will have traveled 400 km and increased the sensitivity 400 times.
A power recycling (PR) cavity comprising the mirrors (PRM, PR2 and PR3) reflects that part of the laser beam going toward the laser back to the beam splitter. To clean the signal coming out of the interferometer and select only the mode sent in a signal recycling (SR) cavity (comprising mirrors SRM, SR2 and SR3) and an output mode cleaner cavity is used.
Most of these mirrors are housed in vacuum chambers and hung in very complex multistage pendula to reduce their coupling to ground motion.
Working on a noisy planet
Because the LIGO detectors are such sensitive instruments, they are easily affected by external disturbances, or what scientists cumulatively call "noise." The dominant noise sources are caused by the very quantum nature of light, thermal vibrations of the atoms making up the optics and their supporting suspensions, and seismic noise from the constant vibration of the Earth's surface.
Other varied effects that can couple to various parts of the interferometer and reduce the instrument sensitivity are man-made ground motion (e.g., trains, cars and trucks); acoustic signals from airplanes; power-supply fluctuations; weather-related events, such as temperature changes, high wind and storms; and many other sources of instrumental and environmental noise.
Stabilizing an instrument that looks for movement 1/10,000 the diameter of a proton is not a simple task. Across each of the LIGO Observatory sites, an extensive array of environment sensors (seismometers, microphones, radio-frequency receivers and temperature sensors) constantly monitor the immediate environment. Thousands of other sensors monitor the state of the instrument and keep it stable ("locked') through computer-actuated, real-time feedback loops and control mechanisms (servo-actuators that hold the mirrors in relation to each other). Data from the components are transmitted in real time to the control room, where LIGO researchers and operators work together to monitor and maintain the total system.
Anamaria Effler, Caltech Postdoctoral Scholar, is working with the Advanced LIGO instrument as it is brought online. "Due to the amazing sensitivity of LIGO, there are noise sources which would never be taken into consideration for other experiments," Effler said. "We have to think what those sources are, try to discover the coupling mechanism, measure the effect, decide if it's a limiting noise source, create a model to explain it and then either reduce the noise of the source or reduce the coupling to the detector. It requires a deep knowledge of many aspects of physics and engineering, so we're always learning."
There are also hurdles in building one of the world's most advanced astronomical observatories. "I dread wind," said Sheila Dwyer, a Massachusetts Institute of Technology postdoctoral researcher at LIGO working with the commissioning team at the Hanford observatory. "The wind can move the ground enough to make things quite difficult. We need to do a lot of work to get to the same point we were when the ground [is] quiet. This is necessary work, because we want to be able to make observations in bad weather as well as good weather."
Now, nearly 30 years after its inception, how will LIGO know if it has met with success? The Advanced LIGO installation is already a success in surpassing its predecessor in sensitivity, just months after its installation.
"We will continue our work to get to the sensitivity we hoped for in the design of the instrument, enabling us to 'see' up to 600 million light-years away," added Effler. Of course, the greatest success will be when we've directly detected a gravitational wave for the first time in human history."
A great leap forward
In 1887, Albert Michelson and Edward Morley, at what is now Case Western Reserve University in Cleveland, Ohio, used a tabletop interferometer to disprove the existence of aether (a then prevalent concept for an invisible medium that propagates light). This Michelson-Morley experiment laid the foundation for Einstein's special and general theories of relativity. Now, LIGO is using kilometer-scale interferometers to directly detect one of Einstein's predictions.
In a letter to scientists Robert Hooke in 1676, Isaac Newton wrote, "If I have seen further it is by standing on the shoulders of giants." Scientific achievements are never the brainchild of a single person, a "lone genius." Rather, they stem from the common work of generations of men and women dedicated to the pursuit of knowledge through the scientific method.
Simple in concept but nearly 30 years in the making, LIGO is built upon one basic principle: the interference of light. But it is the work of many generations of scientists, researchers and engineers who have made possible such an incredible instrument that will soon give us a new way to see the cosmos.
For more on Advanced LIGO, see the film, "LIGO Generations," by Kai Staats. In combination with "LIGO, A Passion for Understanding," these two films provide an insight to the dedication, passion, and incredible challenge that is the LIGO experiment.
Follow all of the Expert Voices issues and debates — and become part of the discussion — on Facebook, Twitter and Google+. The views expressed are those of the author and do not necessarily reflect the views of the publisher. This version of the article was originally published on Space.com.
Join our Space Forums to keep talking space on the latest missions, night sky and more! And if you have a news tip, correction or comment, let us know at: community@space.com.
Kai is an inventor, freelance writer, filmmaker, business developer and member of the MarsCrew134 team who aspires to find expression in the arena of film. He's dedicated to helping humankind become interplanetary while gaining an improved perspective of our role as caretakers for our planet Earth. While not actively engaged in film production he's been capturing the construction of SAM's Biosphere 2 and its use by visiting teams at the University of Arizona research campus near Oracle, Arizona.